28 August 2023: Clinical Research
Impaired Renal Vein Flow in Atrial Fibrillation: A Potential Risk for Renal Dysfunction
Bayram Öztürk
DOI: 10.12659/MSM.941435
Med Sci Monit 2023; 29:e941435
Abstract
BACKGROUND: Atrial fibrillation (AF) is one of the most common heart rhythm disorders. Identification and early treatment of AF risk factors can improve mortality and morbidity rates. This study aimed to compare the renal venous stasis index (RVSI) and intra-renal venous flow (IRVF) patterns evaluated by intra-renal Doppler ultrasonography in patients with AF and sinus rhythm (SR).
MATERIAL AND METHODS: A total of 68 patients, 34 with AF (lasting >12 months AF) and 34 with SR (no previous diagnosis of AF and no AF attack in 24-h Holter monitoring) were included in the study. The RVSI was calculated, and the IRVF patterns were determined using intra-renal Doppler ultrasonography. High RVSI was defined as >0.12 RVSI. In addition, echocardiography and a 6-min walk test were performed. A model including diabetes mellitus, hypertension, creatine, Pro-BNP, left ventricular ejection fraction, presence of AF, and systolic pulmonary artery pressure was created to evaluate the effects of variables on high RVSI.
RESULTS: The RVSI value was significantly higher in patients with AF than in those with SR (P=0.004). The SR group exhibited a higher prevalence of the continuous flow pattern, which is one of the IRVF patterns (P=0.015). In contrast, the biphasic flow pattern was observed more frequently in patients with AF (P=0.003). The presence of AF was found to predict the high RVSI (P=0.002, OR=14.134, 95% CI 2.083-71.277).
CONCLUSIONS: The presence of AF may affect the IRVF and cause an increase in RVSI.
Keywords: Atrial Fibrillation, Cardio-Renal Syndrome, Renal Veins, Ultrasonography, Doppler, Humans, Stroke Volume, Ventricular Function, Left, Kidney Diseases
Background
Atrial fibrillation (AF) is the most common disease with high mortality and morbidity, usually seen in advanced age [1]. The AF can cause a decrease in cardiac output, leading to organ dysfunctions [2]. Severe symptoms can arise from AF in patients with a low left ventricular ejection fraction (LVEF) [3]. The heart and the kidney are 2 closely related organs [4]. While the deterioration of the heart affects renal functions, on the contrary, the decrease in renal functions also impairs the heart [5]. It is necessary to define the risk factors of AF and to clarify its relationship with the kidneys. Old age, hypertension (HT), diabetes mellitus (DM), and structural heart diseases are common risk factors for AF and renal failure [6]. Although there are many studies on renal failure facilitating the development of AF, few studies have been conducted on the role of AF in the occurrence of renal insufficiency [7]. Moreover, most of these studies had an epidemiological research perspective [8].
The kidneys’ anatomical structures and functional status are evaluated by renal Doppler ultrasonography (RDUS) [9], which also allows us to visualize intra-renal arteries and veins [10]. Although the relationship between intra-renal arterial flows and cardiac diseases is well defined, studies on intra-renal venous flow (IRVF) are limited [11]. The IRVF changes have been demonstrated in pre-eclampsia and diabetic nephropathy [10]. However, there is no study showing its relationship with AF. Renal venous stasis index (RVSI) is a novel marker recently used to assess renal congestion status [11]. It is calculated using IRVF time and cardiac cycle time [12]. A correlation between heart failure and RVSI has been reported [11]. In this study, we aimed to evaluate the RVSI and IRVF patterns through intra-renal Doppler ultrasonography in patients with AF and patients with normal sinus rhythm (SR).
Material and Methods
ETHICS STATEMENT:
The study was approved (decision number 2020/11) by the Ethics Committee of Kahramanmaras Sutcu Imam University Faculty of Medicine and complied with the Helsinki Declaration. All participants gave their permission after being fully informed.
STUDY DESIGN:
The study employed an observational and cross-sectional research design. This study included 68 participants who attended the Cardiology outpatient clinic between September 2020 and March 2021. They were divided into 2 groups: a healthy control group (SR) (n=34) and an AF group (n=34). Individuals in the control group had no known AF, and no AF episode could be detected on 24-h Holter monitoring. The AF group consisted of patients evaluated as having long-standing-persistent AF and permanent AF according to the 2020 American AF guideline classification [13]. Criteria for exclusion from the study were having LVEF <50%, prosthetic valve implantation, moderate-to-severe mitral or aortic valve stenosis/regurgitation, glomerular filtration rate <60 mL/min/1.73 m2, coronary artery disease, chronic liver failure, and primary pulmonary hypertension. The demographic characteristics of all participants were noted. Electrocardiography, intra-renal Doppler ultrasonography, and a 6-minute walking test (6MWT) were also performed. The IRVF pattern was determined through intra-renal Doppler ultrasonography, and the RVSI was calculated. Variables were compared between groups.
DEFINITION OF ATRIAL FIBRILLATION:
AF was defined as irregular R-R intervals on the superficial ECG and the absence of prominent recurrent P waves. It was also described as irregular atrial episodes of >30 s on 24-h Holter monitoring. AF classification was based on 2020 American guidelines. Long-standing-persistent AF was defined as AF lasting more than 1 year in which rhythm control strategies were applied. AF lasting more than 1 year without AF ablation and anti-arrhythmic therapy strategies was categorized as permanent AF.
ECHOCARDIOGRAPHIC PARAMETERS:
Echocardiography using Vivid 7 (General Electric, Horten, Norway, 2–4 MHz phased array transducer) was performed on all participants according to American Society of Echocardiology (ASE) and European Society of Cardiovascular Imaging guidelines [14]. It was applied to individuals in the left lateral and decubitus position. Doppler echocardiography and m-mode parameters were measured in 2 dimensions. The LVEF, interventricular septal thickness (IVST), posterior wall thickness (PWT), left ventricular diastolic function, and left ventricular systolic function values were assessed. In addition, tricuspid annular plane systolic excursion (TAPSE) and systolic pulmonary artery pressure (sPAP) were measured. The LVEF biplane was calculated by the Simpson method [14].
DEFINITION OF THE RESISTIVE INDEX AND INTRA-RENAL VENOUS FLOW PATTERNS:
The frequency range was set to 2.5–5.0 MHz using GE LOGIQ E9 (GE Healthcare, Horten, Norway) device for intra-renal Doppler ultrasonography. The IRVF patterns of the patients were measured during breath holding at the end of the expiration. Pulse-colored Doppler waveforms of interlobar arteries and veins were recorded simultaneously in the left lateral decubitus position (Figure 1). Moreover, in Figure 1, the upward Doppler signals indicate the intra-renal arterial flow used to measure the cardiac cycle time. In contrast, a low Doppler signal indicates the venous flow used to measure the venous flow time. The IRVF was divided into 2 groups: continuous (non-congestive) and interrupted (congestive) flow. In interrupted flow models, pulsatile, biphasic (venous peak during systole and diastole), and monophasic (venous peak during diastole) waveforms were categorized. The resistive index (RI) was evaluated in both kidneys, and the mean values of the measurements were calculated. The RI value is calculated as maximum systolic flow velocity - minimum diastolic flow velocity/maximum systolic flow velocity [9]. All RDUS tests were performed in the right kidney. The rationale behind using the right kidney was that since the left ovarian or testicular venous vessels empty directly into the left renal vein, the IRVF might be affected if these vessels expand or contract between large veins.
DESCRIPTION OF RENAL VENOUS STASIS INDEX:
The RVSI is obtained by dividing the period of absence of IRVF by the total duration of the cardiac cycle through intra-renal Doppler ultrasonography (RVSI = cardiac cycle (ms) – venous flow (ms)/cardiac cycle (ms)) (Figure 2). In patients with SR, the average of at least 3 measurements obtained from interlobar vessels during the cardiac cycle was calculated. In patients with AF, it was calculated using 1 cardiac cycle for each measurement (beats following the previous 2 cardiac cycles of equal duration) [10]. In addition, the patients were divided into 4 groups considering their RVSI values: RVSI=0, 0< RVSI ≤0.12, 0.12< RVSI ≤0.32, and RVSI >0.32. In addition, RVSI was also categorized into 2 groups: low (RVSI ≤0.12) and high RVSI (>0.12).
STATISTICAL ANALYSIS:
All statistical analyses were performed using SPSS v. 22.0 (IBM, Inc., USA). Continuous variables were reported as mean±standard deviation and categorical variables were reported as percentages. Data normality was checked by using the Kolmogorov-Smirnov test. Skewed data were expressed as the median with the interquartile range. The independent-samples
Results
DEMOGRAPHIC DATA:
The baseline parameters of the patients are shown in Table 1. When the baseline parameters of both groups were examined, the distribution of age, gender, HT, DM, chronic obstructive pulmonary disease, chronic kidney disease, and smoking in both groups was similar. Although the average age of the patients with AF was higher than those with SR, there was no statistically significant difference between the 2 groups (62.14±9.56 and 58.62±7.80, respectively, P=0.108).
ECHOCARDIOGRAPHIC AND BIOCHEMICAL DATA:
The echocardiography parameters of the patients are shown in Table 2. Of the echocardiography parameters, right atrial (RA) diameter, IVST, PWT, left atrial (LA) diameter, left ventricular end-systolic diameter, and end-diastolic diameter had similar values in both groups. It was observed that the values of RA area (P<0.001), RV area (P=0.001), and sPAP (P<0.001) were higher in the AF group than those of the SR group. However, TAPSE, one of the indicators of right ventricular function, was lower in the AF group (P<0.001). Also, the LA diameter (P=0.001) and LA area (P<0.001) values were higher in the AF group than in the SR group. LVEF was significantly lower in patients with AF than in patients with SR (P=0.001).
Table 3 shows that when the laboratory parameters of the groups were examined, it was found that BUN (P=0.011) and Pro-BNP (P=0.032) levels of patients with AF were significantly higher than those of patients with SR. In addition, albumin levels were lower in patients with AF than in those with SR (P=0.001).
INTRA-RENAL DOPPLER ULTRASONOGRAPHY:
Table 4 shows the RDUS parameters, in which there was no significant difference in RI value between the 2 groups (P=0.240). On the other hand, the RVSI value was significantly higher in patients with AF compared to those with SR: median 0.20 (0.00–0.38) and 0.00 (0.00–0.10), respectively (P=0.004). Regarding the IRVF parameters, continuous flow was more common in patients with SR (n=22; 64.7%), and a statistically significant difference was observed between the groups (P=0.015). However, the pulsatile flow pattern showed similar distribution between the groups (P=0.798). On the other hand, the biphasic flow pattern was more common in patients with AF (P=0.003). The lowest rate of biphasic flow pattern and the highest rate of continuous flow pattern was observed in patients with SR (2.9%, and 64.7%, respectively). The highest biphasic flow pattern was observed in patients with AF, and the number of patients with continuous and pulsatile flow patterns was the same (29.4% and 35.3%, respectively).
CLINICAL PARAMETERS OF RVSI TERTILES:
We assessed and compared the clinical and laboratory parameters according to RVSI groups (Table 5). In the fourth RVSI group, the number of female patients was higher than male patients. In both groups of patients with AF and SR, the highest number of patients was seen in the first RVSI group. There was a significant difference between the 6MWT distance in RVSI groups (P=0.002). The shortest 6MWT distance was observed in the fourth RVSI group (289.52±72.16 m). Pro-BNP levels were the highest in the third RVSI group (820.18±160.56 ng/L) and the lowest in the first RVSI group (552.44±101.56 ng/L). Table 6 shows the variables included in the logistic regression analysis affecting the high RVSI. The presence of AF increased the risk of high RVSI 14.13 times.
Discussion
In this study, we showed that AF could affect IRVF patterns independently of pulmonary artery pressure, and recently, a high RVSI, a new indicator of renal congestion, was found to be associated with AF. We monitored continuous and biphasic flow patterns of IRVF in patients with AF. We found that the 6MWT decreased as the RVSI stage increased. Right and left heart chambers were larger in patients with AF. Finally, we found AF was an independent indicator of high RVSI.
AF is irregular contractions in the atrium, in which dilatation of the atriums and ventricles may occur, and the blood may pool behind due to loss of contractile functions of the atriums. As a result of increased intracardiac pressure, the returning blood flow augments the interstitial and tubular hydrostatic pressure in the kidney, which is surrounded by the capsule. At the same time, the deterioration in left heart function increases pulmonary vascular resistance and worsens right heart function. AF causes a decrease in cardiac output and an increase in central venous pressure (CVP). The increase in CVP is reflected in the renal veins, resulting in congestion [15,16], and increased congestion increases renal venous pressure.
Additionally, it leads to decreased renal perfusion pressure, which reflects the difference between renal arterial and venous pressure. Renal perfusion pressure is an essential determinant of glomerular filtration rate. Decreased glomerular perfusion pressure due to AF reduces the glomerular filtration rate and causes deterioration in kidney function [17]. This condition is known as cardio-renal syndrome [18]. In our study, systolic sPAP elevation was not detected in the AF group, although enlargement was observed in the cardiac structures. This is because although echocardiography is sufficient to measure SPAP, it cannot replace the criterion standard right heart catheterization. The deterioration of IRVF in AF can be explained by pathophysiological mechanisms, similar to cardio-renal syndrome. However, AF affects IRVF without a high sPAP level, and unidentified inflammatory processes may play a role.
Determining the degree of congestion or the type of venous flow pattern is paramount to understanding renal dysfunction [19]. In the present study, the continuous flow pattern was the most common among patients with SR, and the biphasic flow pattern was most common in patients with AF. As the severity and duration of cardiac diseases increase, the IRVF deteriorates. The IRVF is valuable as it reflects the relationship between cardiovascular diseases and the kidney [20]. One of the more sensitive parameters obtained from IRVF patterns in recent years is the RVSI. It shows the severity of renal congestion. The RVSI is a constant value according to IRVF patterns. A study concluded that RVSI is superior to IRVF in determining the relationship between cardiovascular diseases and renal congestion [12]. Because there is a continuous venous flow in the kidney under physiological conditions, a continuous flow (RVSI=0) is observed. However, when the venous flow deteriorates in favor of congestion, this continuity disappears, and the RVSI value increases (RVSI >0). Clinically, an elevated RVSI index may result in increased heart failure staging and renal dysfunction. Demonstrating IRVF at an early stage without elevation of creatinine may help provide treatment at an early stage [21]. In our study, IRVF patterns and RVSI values supported renal congestion in patients with AF. These parameters may allow us to categorize the patients to the LVEF-preserved heart failure group and to use them in the classification of heart failure.
The heart and kidneys are essential for maintaining cardiovascular hemodynamics. These processes depend on many complex neurohormonal balances between the heart and kidney. Neurohormonal balance is regulated by the renin-angiotensin-aldosterone system (RAAS), sympathetic nervous system, and atrial natriuretic peptides. It does not cause renal dysfunction only by creating hemodynamic congestion in the kidney; the pertinent literature shows additional evidence that renal damage occurs in various ways. First, activation of the RAAS in patients with AF causes left ventricular hypertrophy, myocardial fibrosis, left atrial enlargement, and increased intracardiac pressure. At the same time, RAAS can cause direct renal damage, particularly in nephrons [22]. Second, systemic inflammation results in intracardiac structural changes and the emergence and persistence of AF. AF induces intracardiac fibrosis, so the same fibrotic process can occur in the kidneys. Therefore, a systemic fibrotic process begins in patients with AF [23]. Third, the deterioration of renal vascular endothelial functions and increased prothrombotic status in AF patients trigger platelet activation. Similar to silent cerebral microinfarcts seen in patients with AF, renal microinfarcts occur and cause kidney function deterioration [24].
Navaravong et al reported improvement in renal function in patients undergoing SR after AF ablation compared to pre-ablation [25]. Hunter et al showed increased LVEF in AF ablation patients [26]. Controlling chronic systemic diseases (eg, DM and HT) is the primary treatment strategy in chronic kidney disease. In our study, DM and HT rates were similar in both groups. However, case-control studies are needed to compare IRVF between patients with systemic diseases and healthy patients.
This study has some limitations. First, due to the invasiveness of tests evaluating the cardiac effects of AF, right heart catheterization was not performed, and left ventricular end-diastolic pressure was not measured. Second, we did not find a monophasic flow pattern, although it was not one of the exclusion criteria in our study. This study included patients with LVEF >50%. If patients with AF and heart failure were included, we would not see the effect of AF on IRVF patterns. Third, our study covered a relatively small population. Cohort studies are needed to better evaluate the cause-effect relationship. In addition, the IRVF and RVSI parameters may change AF classification in the future and expand the indication for AF ablation.
Conclusions
The presence of AF was a predictor of high RVSI. In addition, patients with AF had worse IRVF patterns. Finally, in the long-term follow-up of patients with AF, evaluation with renal Doppler ultrasonography may show deterioration in renal functions in the early period.
Figures
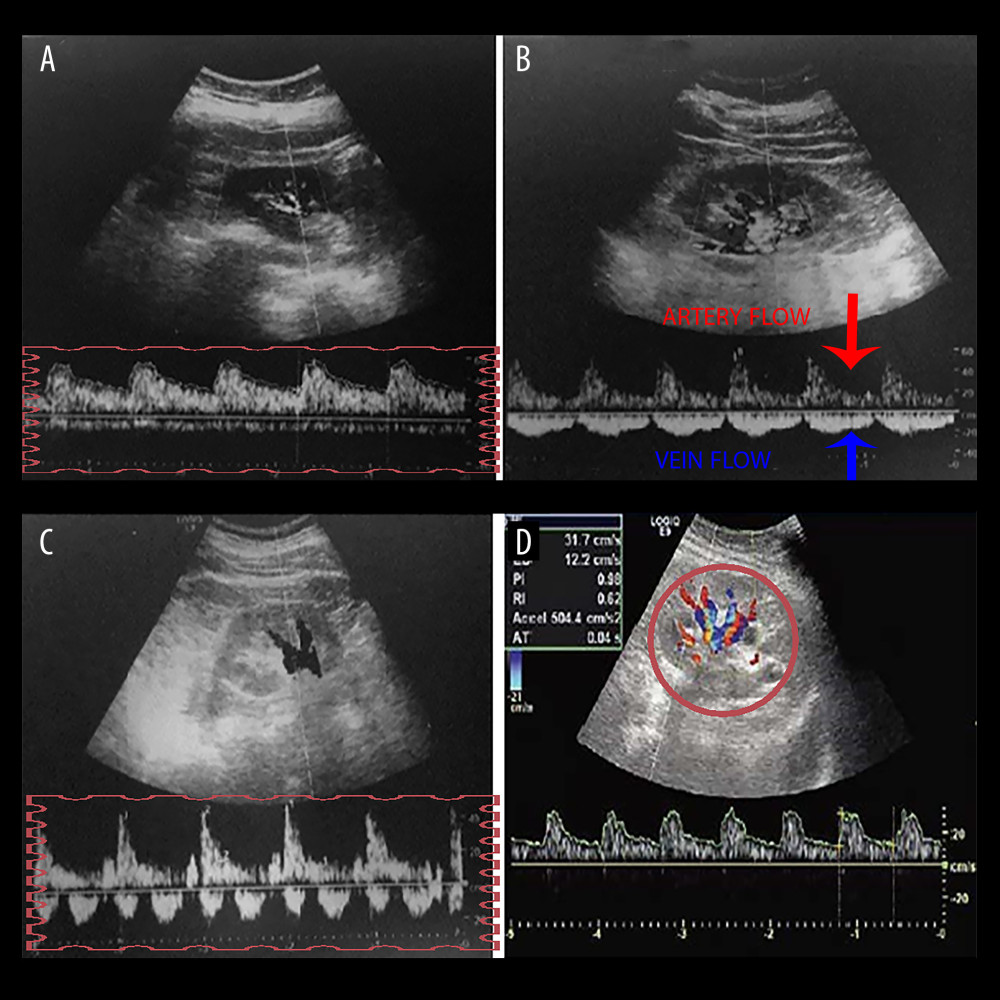
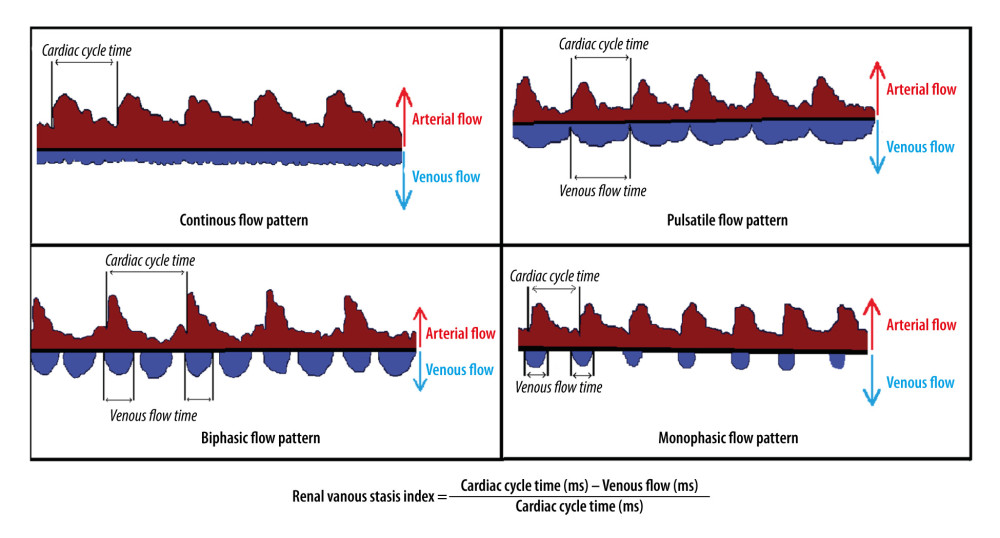
Tables
Table 1. Baseline characteristics of groups.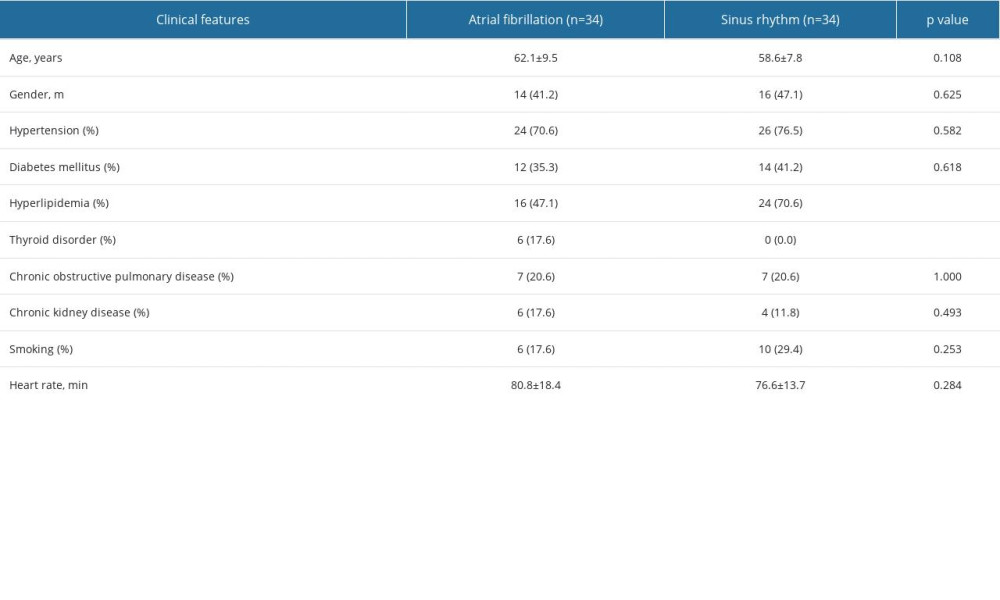
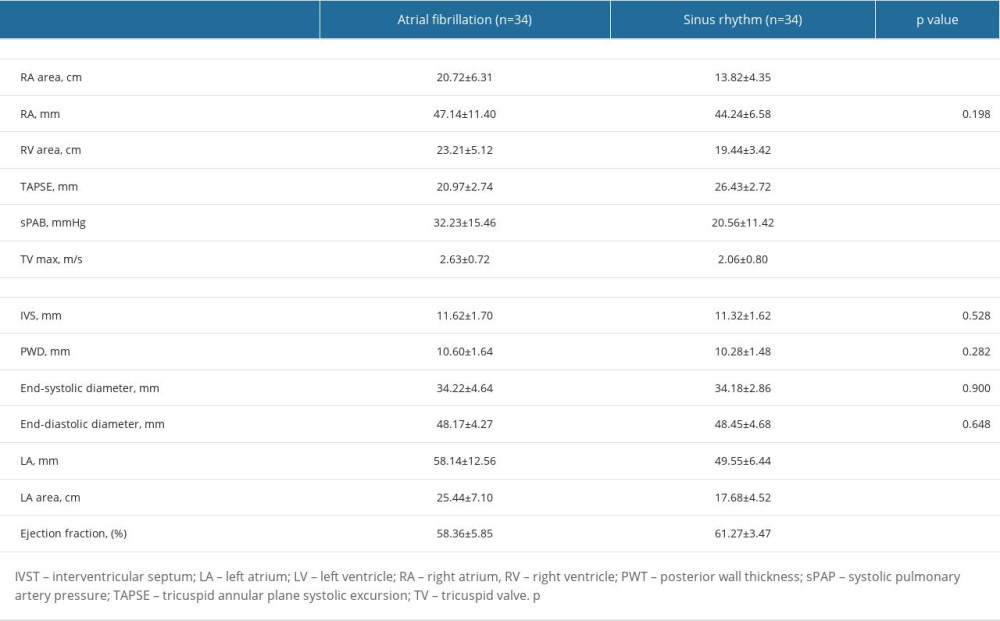
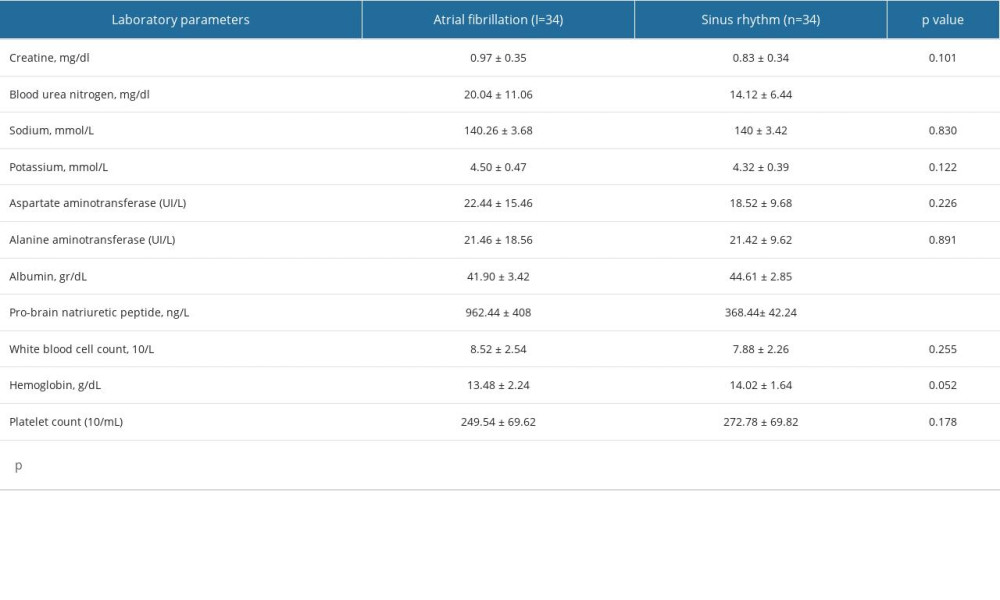
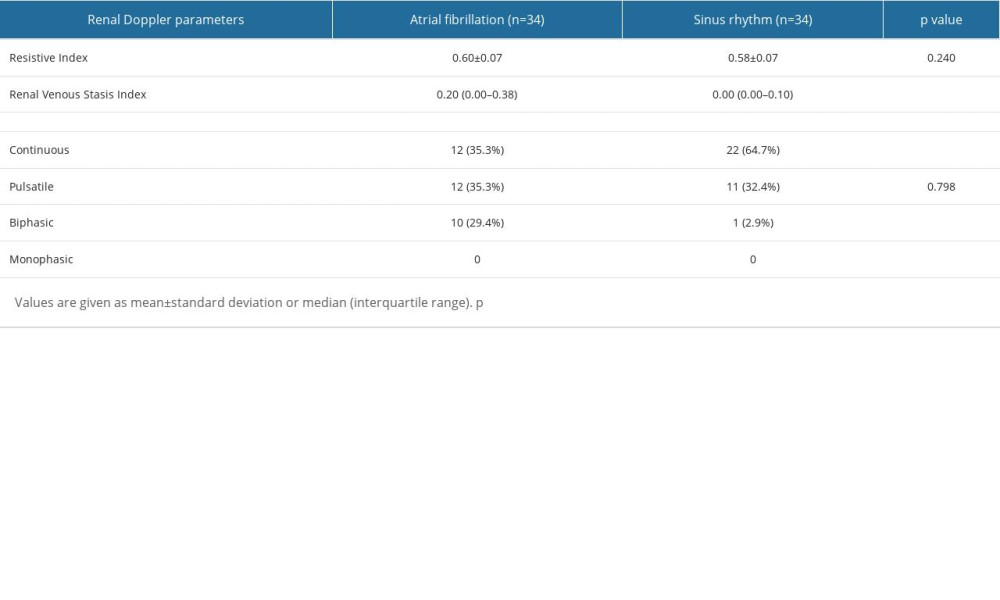
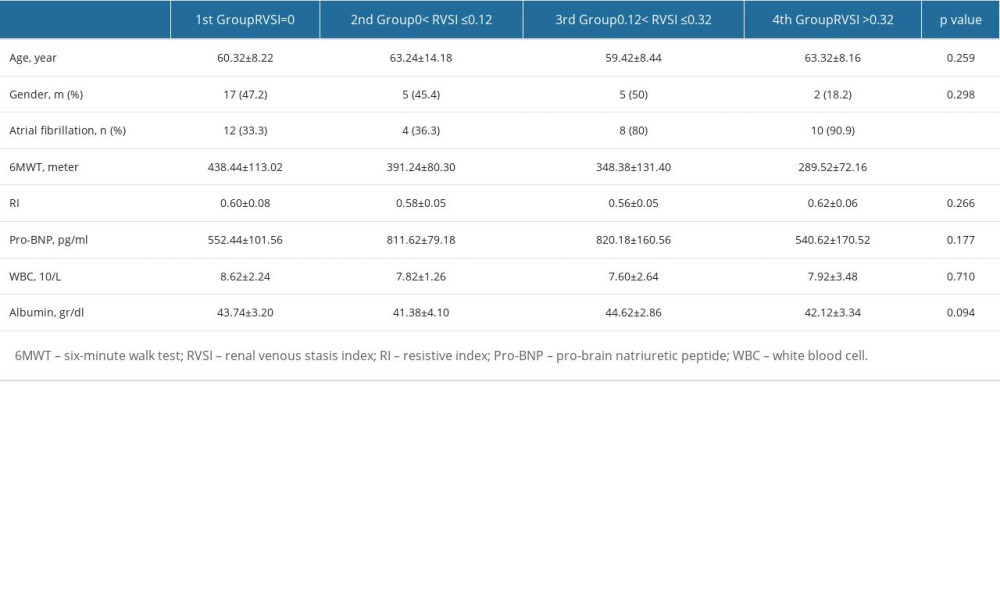
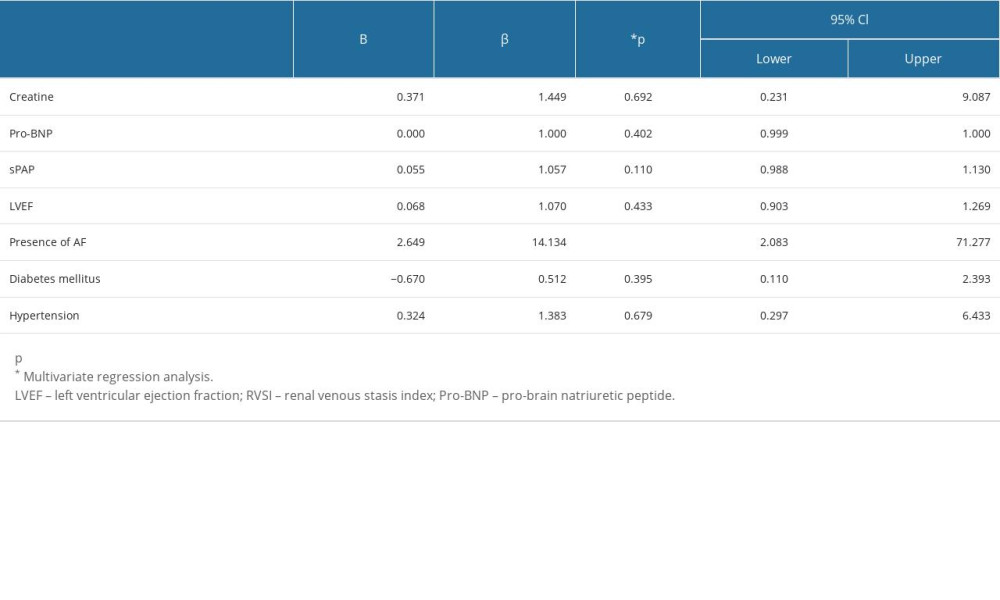
References
1. Chugh SS, Havmoeller R, Narayanan K, Worldwide epidemiology of atrial fibrillation: A Global Burden of Disease 2010 Study: Circulation, 2014; 129(8); 837-47
2. Reddy Y, Obokata M, Verbrugge F, Atrial dysfunction in patients with heart failure with preserved ejection fraction and atrial fibrillation: J Am Coll Cardiol, 2020; 76(9); 1051-64
3. Carrero JJ, Trevisan M, Evans M, Kidney function and the risk of heart failure in patients with new-onset atrial fibrillation: Int J Cardiol, 2020; 320; 101-5
4. Watanabe H, Watanabe T, Sasaki S, Close bidirectional relationship between chronic kidney disease and atrial fibrillation: The Niigata preventive medicine study: Am Heart J, 2009; 158(4); 629-36
5. Takahashi Y, Takahashi A, Kuwahara T, Renal function after catheter ablation of atrial fibrillation: Circulation, 2011; 124(22); 2380-87
6. Major RW, Cheng MRI, Grant RA, Cardiovascular disease risk factors in chronic kidney disease: A systematic review and meta-analysis: PLoS One, 2018; 13(3); e0192895
7. Go AS, Chertow GM, Fan D, Chronic kidney disease and the risks of death, cardiovascular events, and hospitalization: N Engl J Med, 2004; 351(13); 1296-305
8. Genovesi S, Pogliani D, Faini A, Prevalence of atrial fibrillation and associated factors in a population of long-term hemodialysis patients: Am J Kidney Dis, 2005; 46(5); 897-902
9. Singla RK, Kadatz M, Rohling R, Kidney ultrasound for nephrologists: A review: Kidney Med, 2022; 4(6); 100464
10. Jeong SH, Jung DC, Kim SH, Renal venous doppler ultrasonography in normal subjects and patients with diabetic nephropathy: Value of venous impedance index measurements: J Clin Ultrasound, 2011; 39(9); 512-18
11. Iida N, Seo Y, Sai S, Clinical implications of intrarenal hemodynamic evaluation by Doppler ultrasonography in heart failure: JACC Heart Fail, 2016; 4(8); 674-82
12. Husain-Syed F, Birk HW, Ronco C, Doppler-derived renal venous stasis index in the prognosis of right heart failure: J Am Heart Assoc, 2019; 8(21); e013584
13. Hindricks G, Potpara T, Dagres N, 2020 ESC Guidelines for the diagnosis and management of atrial fibrillation developed in collaboration with the European Association for Cardio-Thoracic Surgery (EACTS): The Task Force for the diagnosis and management of atrial fibrillation of the European Society of Cardiology (ESC) Developed with the special contribution of the European Heart Rhythm Association (EHRA) of the ESC: Eur Heart J, 2021; 42(5); 507
14. Mitchell C, Rahko PS, Blauwet LA, Guidelines for performing a comprehensive transthoracic echocardiographic examination in adults: Recommendations from the american society of echocardiography: Am J Kidney Dis, 2019; 32(1); 1-64
15. Hsu LF, Jaïs P, Sanders P, Catheter ablation for atrial fibrillation in congestive heart failure: N Engl J Med, 2004; 351(23); 2373-83
16. Manning WJ, Silverman DI, Katz SE, Impaired left atrial mechanical function after cardioversion: Relation to the duration of atrial fibrillation: J Am Coll Cardiol, 1994; 23(7); 1535-40
17. Collard D, van Brussel PM, van de Velde L, Estimation of intraglomerular pressure using invasive renal arterial pressure and flow velocity measurements in humans: J Am Soc Nephrol, 2020; 31(8); 1905-14
18. Goffredo G, Barone R, Di Terlizzi V, Biomarkers in cardiorenal syndrome: J Clin Med, 2021; 10(15); 3433
19. Gnanaraj JF, von Haehling S, Anker SD, The relevance of congestion in the cardio-renal syndrome: Kidney Int, 2013; 83(3); 384-91
20. Braam B, Cupples WA, Joles JA, Systemic arterial and venous determinants of renal hemodynamics in congestive heart failure: Heart Fail Rev, 2012; 17(2); 161-75
21. Husain-Syed F, Birk HW, Tello K, Alterations in Doppler-derived renal venous stasis index during recompensation of right heart failure and fluid overload in a patient with pulmonary hypertension: Rev Cardiovasc Med, 2019; 20(4); 263-66
22. He X, Gao X, Peng L, Atrial fibrillation induces myocardial fibrosis through angiotensin II type 1 receptor-specific Arkadia-mediated downregulation of Smad7: Circ Res, 2011; 108(2); 164-75
23. Chung MK, Martin DO, Sprecher D, C-reactive protein elevation in patients with atrial arrhythmias: Inflammatory mechanisms and persistence of atrial fibrillation: Circulation, 2001; 104(24); 2886-91
24. Das RR, Seshadri S, Beiser AS, Prevalence and correlates of silent cerebral infarcts in the Framingham offspring study: Stroke, 2008; 39(11); 2929-35
25. Navaravong L, Barakat M, Burgon N, Improvement in estimated glomerular filtration rate in patients with chronic kidney disease undergoing catheter ablation for atrial fibrillation: J Cardiovasc Electrophysiol, 2015; 26(1); 21-27
26. Hunter RJ, Berriman TJ, Diab I, A randomized controlled trial of catheter ablation versus medical treatment of atrial fibrillation in heart failure (the CAMTAF trial): Circ Arrhythm Electrophysiol, 2014; 7(1); 31-38
Figures
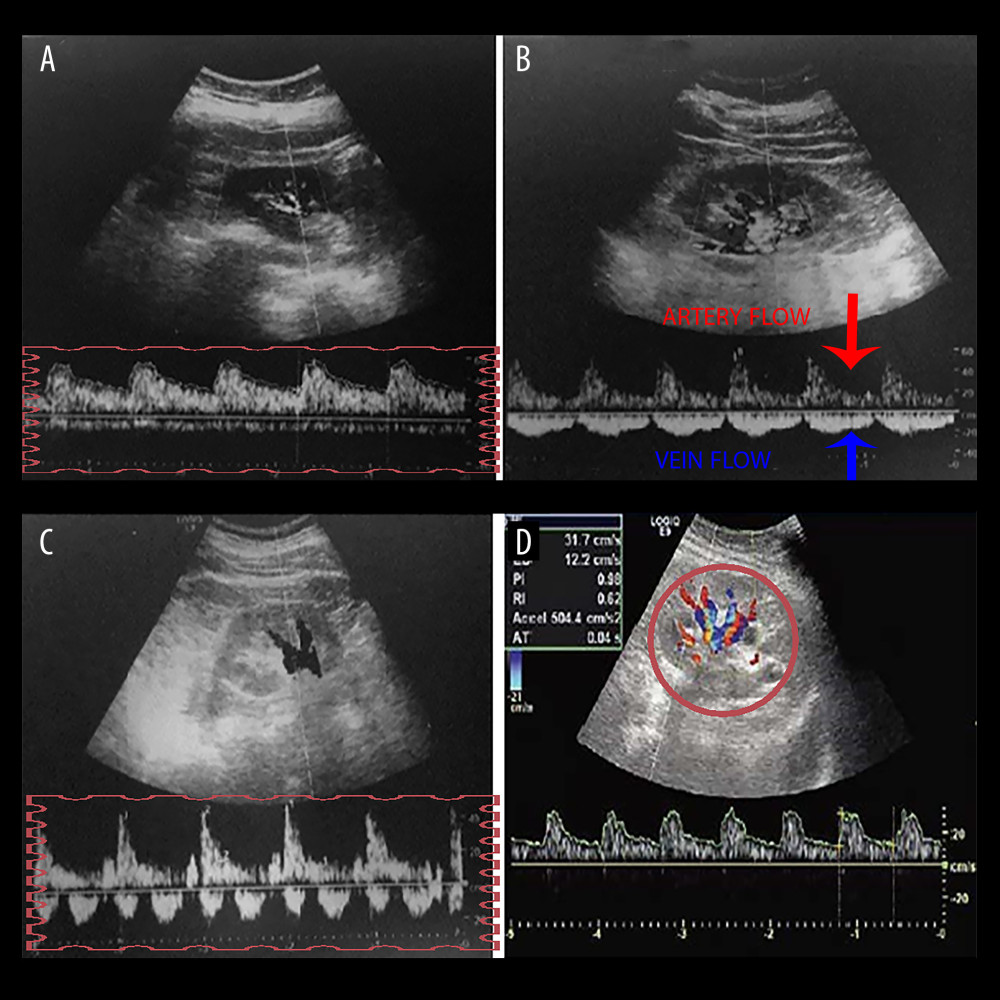
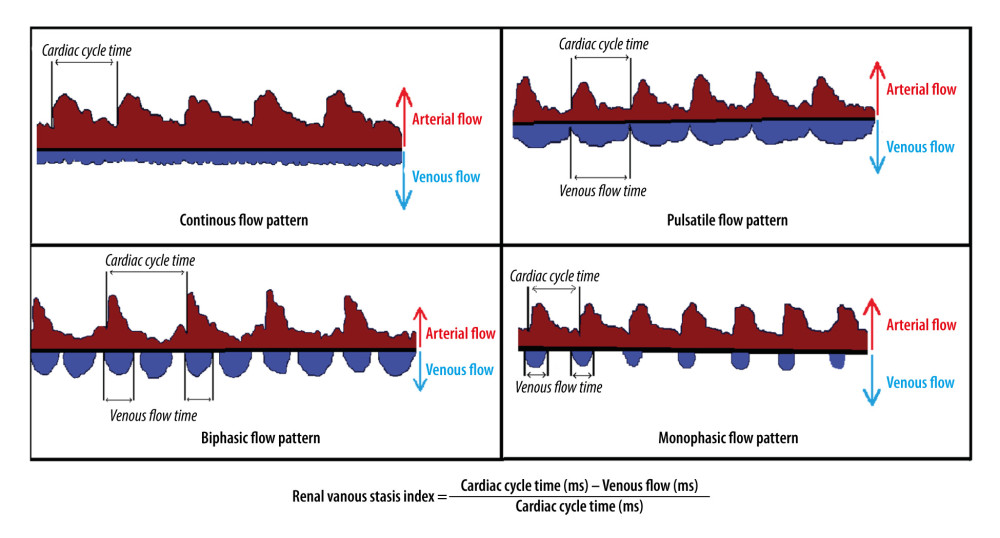
Tables
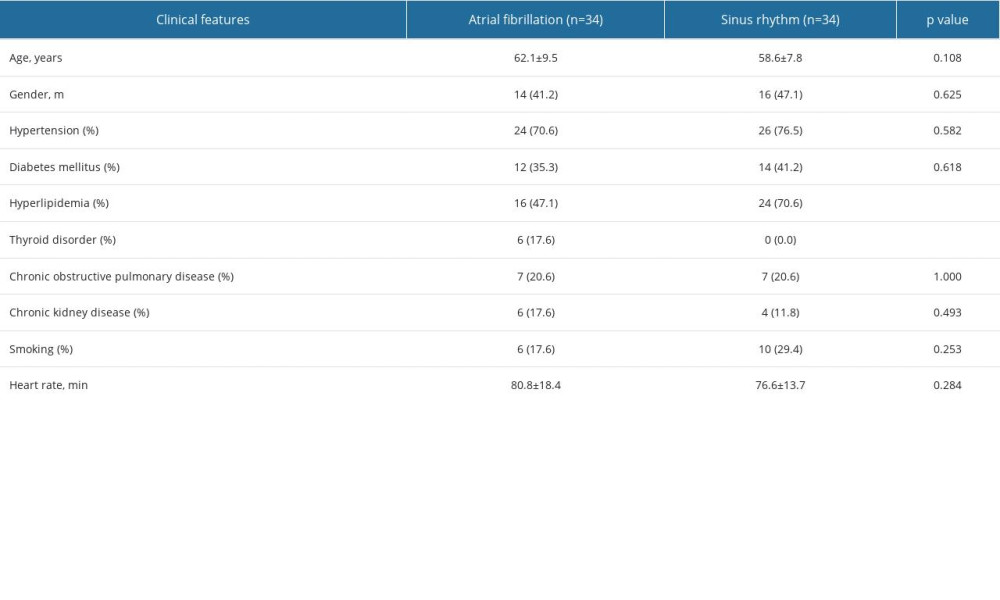
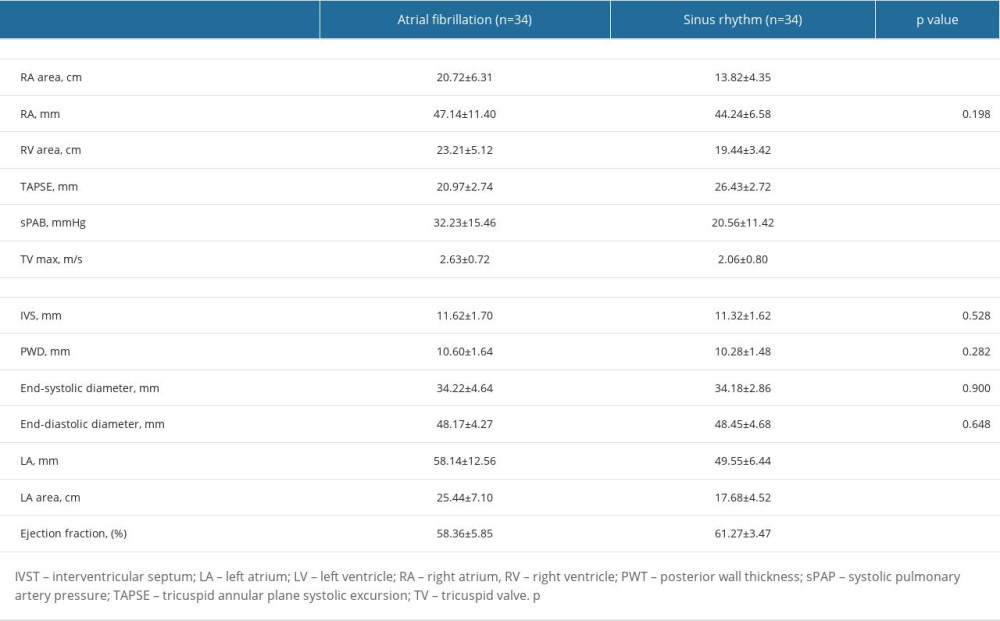
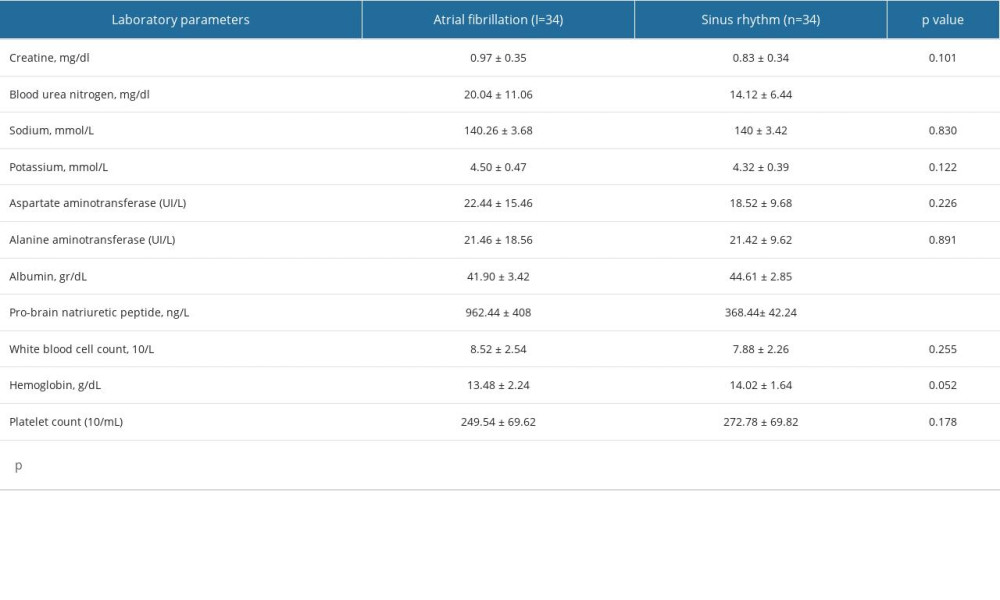
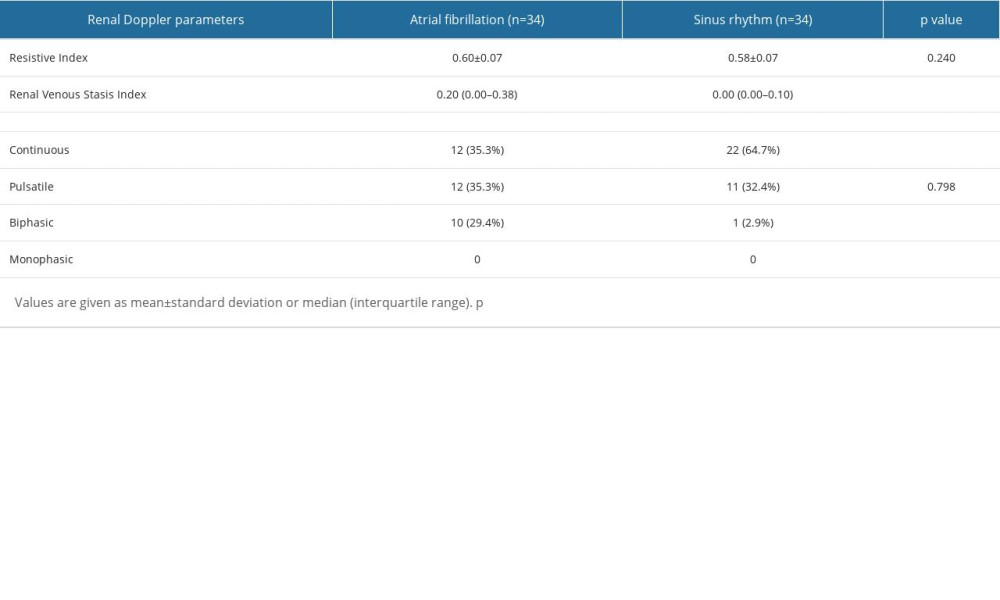
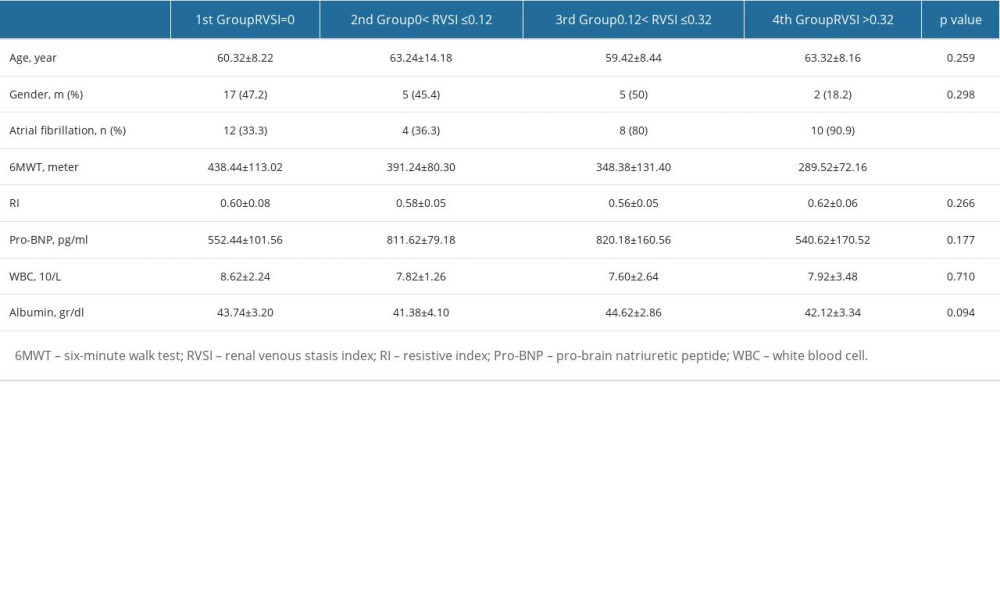
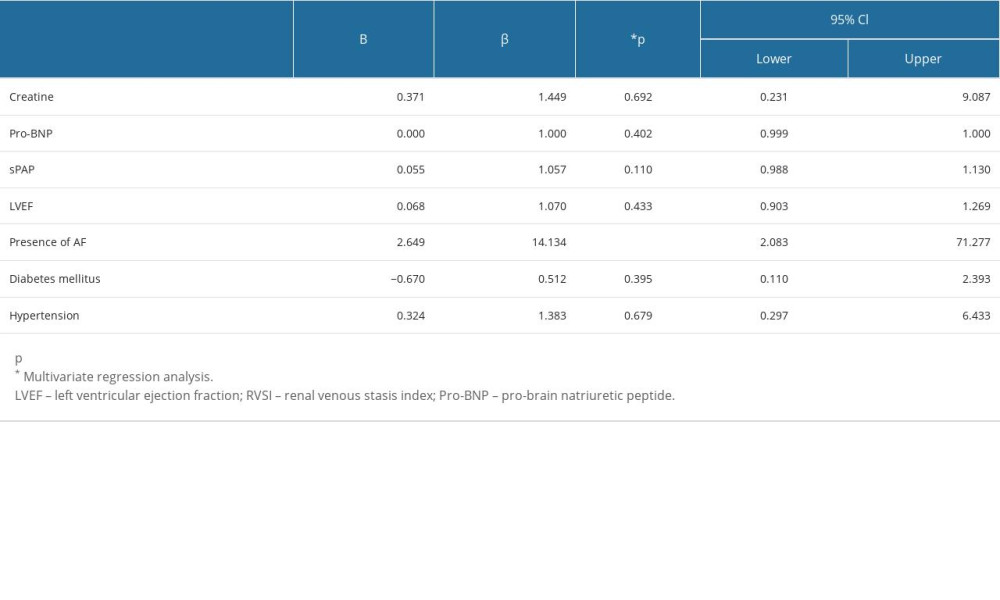
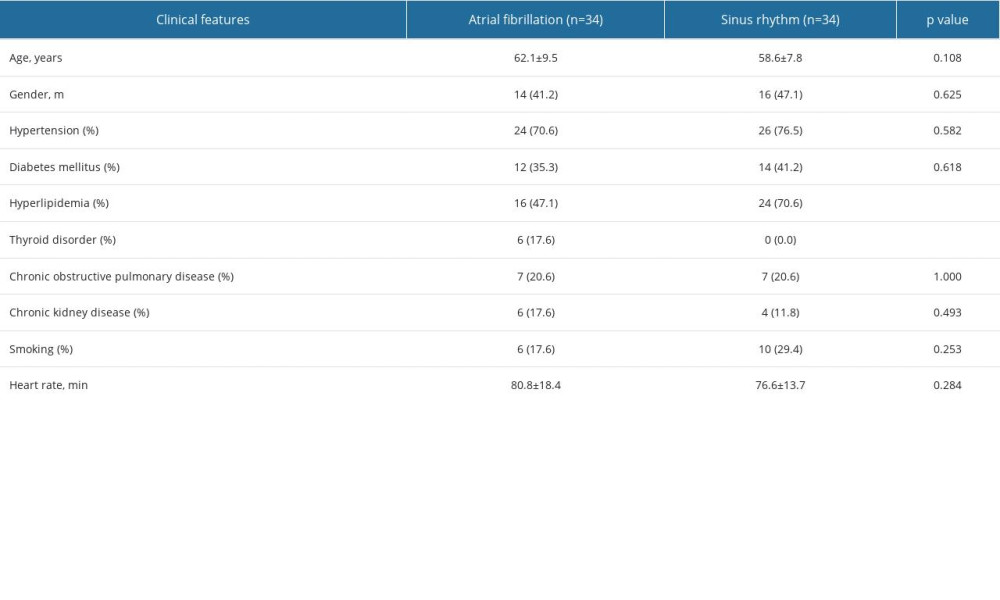
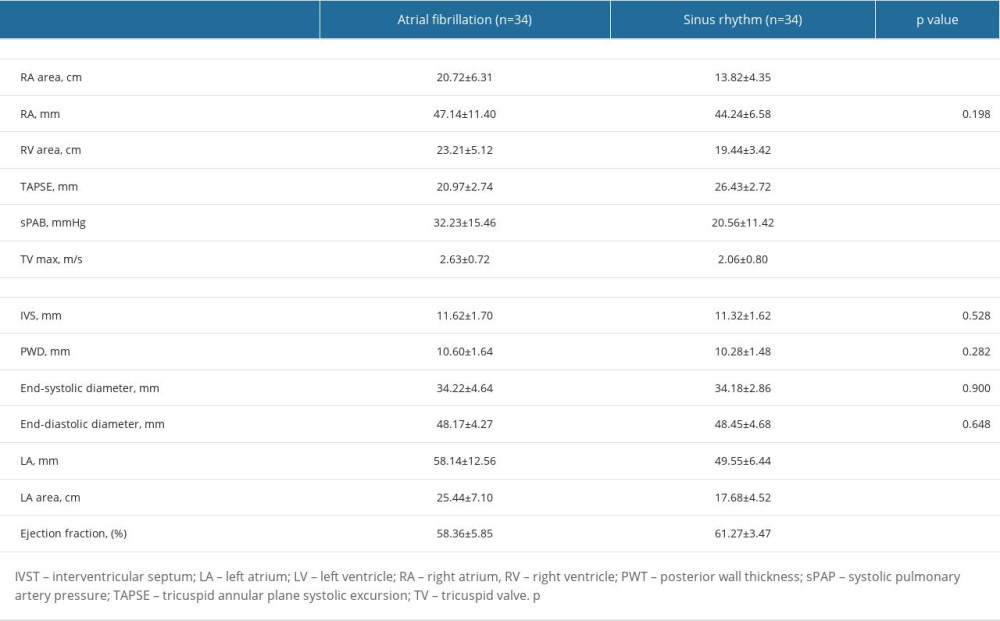
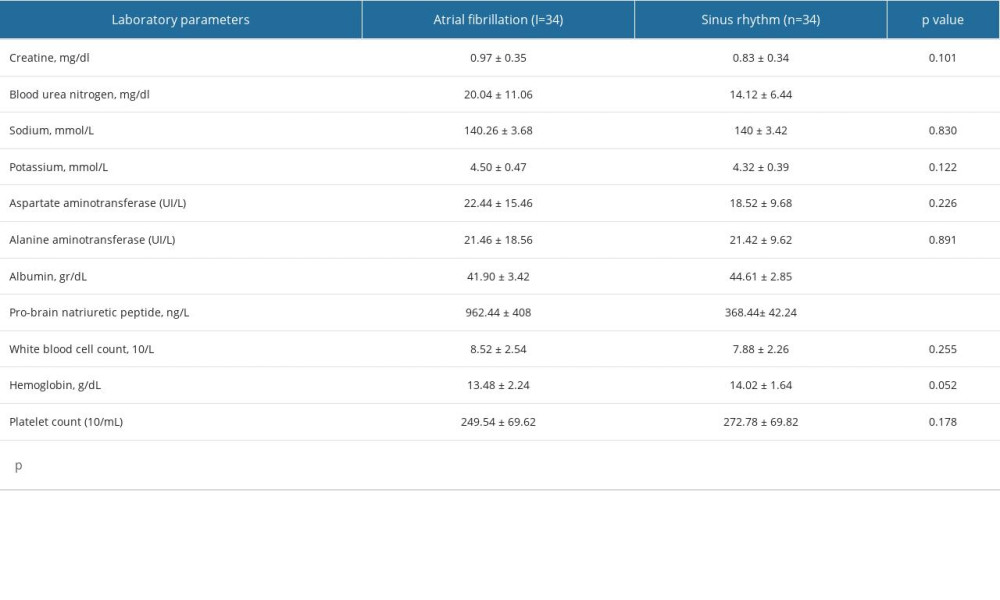
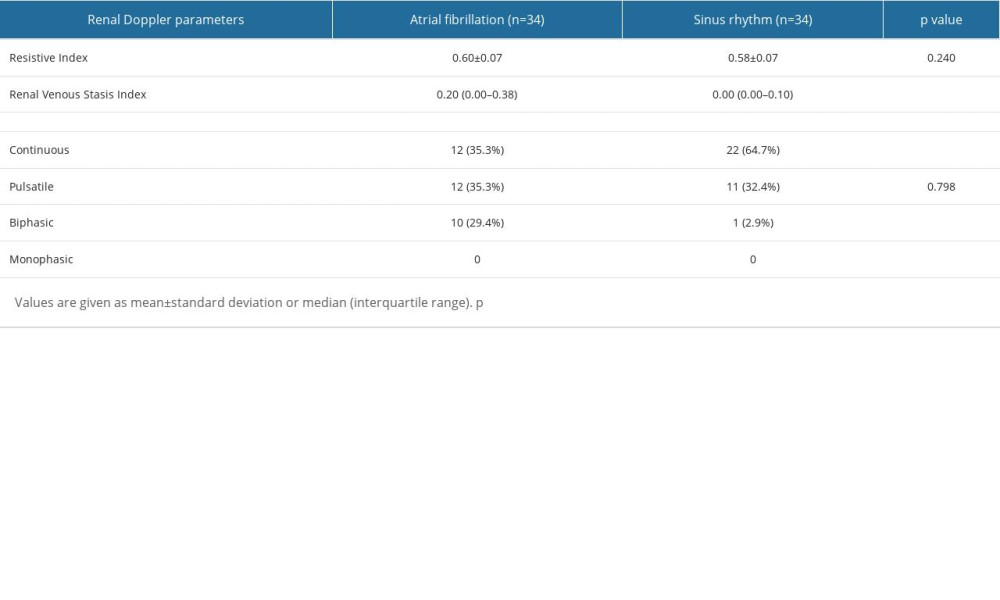
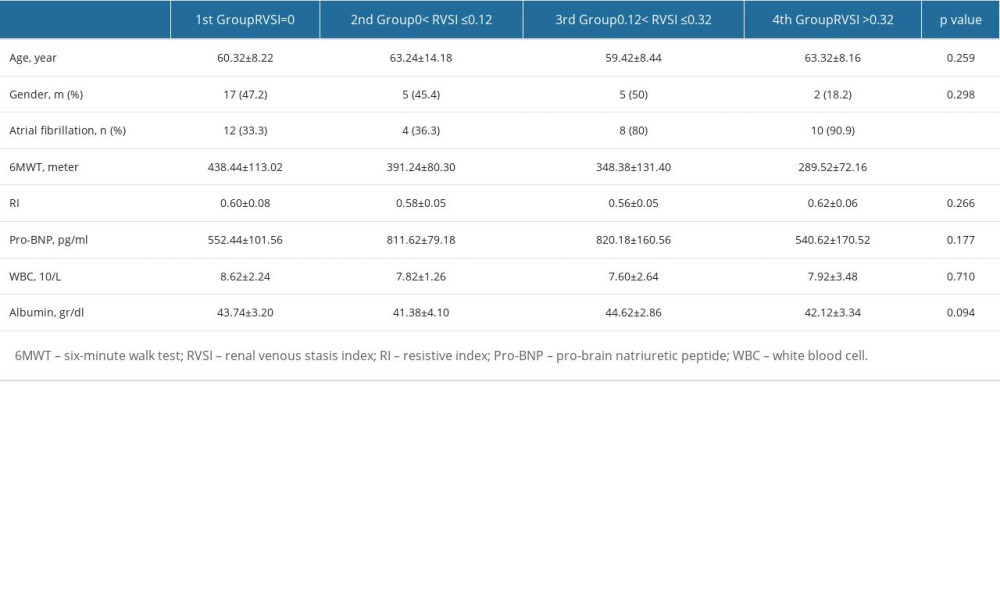
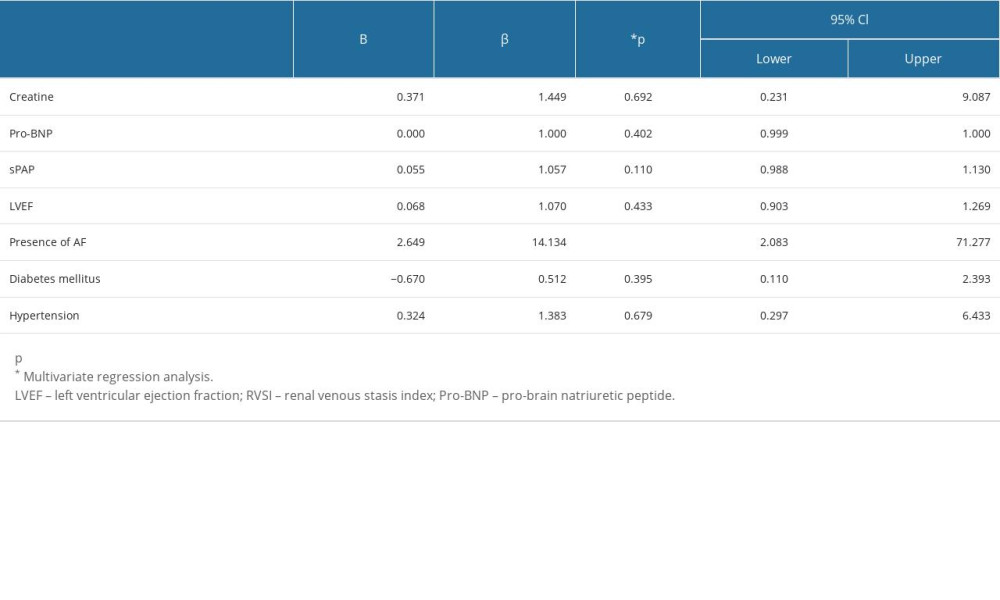
In Press
12 Mar 2024 : Clinical Research
Metabolomic Alterations in Methotrexate Treatment of Moderate-to-Severe PsoriasisMed Sci Monit In Press; DOI: 10.12659/MSM.943360
14 Mar 2024 : Clinical Research
Renal Dysfunction Increases Risk of Adverse Cardiovascular Events in 5-Year Follow-Up Study of Intermediate...Med Sci Monit In Press; DOI: 10.12659/MSM.943956
15 Mar 2024 : Clinical Research
Impact of One-Lung Ventilation on Oxygenation and Ventilation Time in Thoracoscopic Heart Surgery: A Compar...Med Sci Monit In Press; DOI: 10.12659/MSM.943089
14 Mar 2024 : Clinical Research
Differential DHA and EPA Levels in Women with Preterm and Term Births: A Tertiary Hospital Study in IndonesiaMed Sci Monit In Press; DOI: 10.12659/MSM.943895
Most Viewed Current Articles
17 Jan 2024 : Review article
Vaccination Guidelines for Pregnant Women: Addressing COVID-19 and the Omicron VariantDOI :10.12659/MSM.942799
Med Sci Monit 2024; 30:e942799
14 Dec 2022 : Clinical Research
Prevalence and Variability of Allergen-Specific Immunoglobulin E in Patients with Elevated Tryptase LevelsDOI :10.12659/MSM.937990
Med Sci Monit 2022; 28:e937990
16 May 2023 : Clinical Research
Electrophysiological Testing for an Auditory Processing Disorder and Reading Performance in 54 School Stude...DOI :10.12659/MSM.940387
Med Sci Monit 2023; 29:e940387
01 Jan 2022 : Editorial
Editorial: Current Status of Oral Antiviral Drug Treatments for SARS-CoV-2 Infection in Non-Hospitalized Pa...DOI :10.12659/MSM.935952
Med Sci Monit 2022; 28:e935952