01 February 2024: Animal Study
Effects of Intrathecal Ketamine on Cerebrospinal Fluid Levels of Brain-Derived Neurotrophic Factor and Mechanical Allodynia in a Rat Model of Mild Traumatic Brain Injury
Jiyoun Lee








DOI: 10.12659/MSM.942574
Med Sci Monit 2024; 30:e942574
Abstract
BACKGROUND: Ketamine, a compelling candidate for neuropathic pain management, has attracted interest for its potential to elevate brain-derived neurotrophic factor (BDNF) levels. We aimed to assess the effects of intrathecally administered ketamine on the cerebrospinal fluid (CSF) levels of BDNF(c-BDNF) and allodynia in a rat model of traumatic brain injury (TBI).
MATERIAL AND METHODS: Forty-five rats were divided into 3 groups: sham operation (Group S), untreated TBI (Group T), and ketamine-treated TBI (Group K), with 15 rats in each group. Rats were anesthetized, and their skulls were secured in a stereotactic frame before undergoing craniotomy. A controlled cortical impact (CCI) was induced, followed by injection of ketamine (3.41 µg/g) into the CSF in Group K. In Group T, no drug was injected after CCI delivery. On postoperative days (POD) 1, 7, and 14, the 50% mechanical withdrawal threshold (50% MWT) and c-BDNF levels were assessed.
RESULTS: Groups T and K exhibited a significantly lower 50% MWT than Group S on POD 1(6.6 [5.7, 8.7] g, 10.0 [6.8, 11.6] g, and 18.7 [11.6, 18.7] g, respectively; P<0.001). The c-BDNF levels in Group K were significantly higher than those in Groups S and T on POD 1 (18.9 [16.1, 23.0] pg/ml, 7.3 [6.0, 8.8] pg/ml, and 11.0 [10.6, 12.3] pg/ml, respectively; P=0.006).
CONCLUSIONS: Intrathecal ketamine administration did not exhibit anti-allodynic effects following mild TBI. c-BDNF level is a promising potential indicator for predicting the expression of allodynia after mild TBI.
Keywords: Brain Injuries, Traumatic, Brain-Derived Neurotrophic Factor, Cerebrospinal Fluid, Hyperalgesia, Ketamine
Background
With a prevalence of 106 per 100 000 individuals worldwide, traumatic brain injury (TBI) has a relatively high incidence that is increasing annually [1]. As the survival rate of patients with TBI has improved substantially owing to breakthroughs in medical technology and treatment strategies, the focus has shifted from solely providing short-term treatment to the long-term rehabilitation and social reintegration of survivors of TBI [2].
Neuropathic pain is a prevalent concern among survivors of TBI, with approximately two-thirds of them reporting discomfort associated with neuropathic pain [3]. Unlike acute pain, TBI-induced neuropathic pain does not correlate with the severity of the initial tissue injury [4]. Allodynia, one of the prevalent manifestations of neuropathic pain, is characterized by pain triggered by a stimulus that ordinarily would not provoke pain under normal conditions [5,6]. Clinically, allodynia is defined as pain is induced by mild contact that would not normally cause pain, indicating a decrease in the pain sensitivity threshold. Consistent with prior research findings, it has been established that mechanical allodynia can manifest as a sequela following TBI [7].
Recent research has provided evidence supporting the hypothesis that neurotrophins released during neuroprotection and neuro-regeneration following neuronal damage can induce neuropathic pain, manifesting as allodynia or hyperalgesia [8].
The N-methyl-D-aspartate (NMDA) receptor of the L-glutamate receptor family is essential for synaptic transmission, neuroplasticity, and memory storage. In neuropathic pain, dysfunctional NMDA receptors can contribute to central sensitization, amplifying pain signaling and leading to chronic pain [9,10]. Ketamine, as a noncompetitive antagonist of NMDA and glutamate receptors, and a partial agonist of the Mu opioid receptor, has dissociative anesthetic and analgesic effects [11]. Ketamine also has been extensively studied as a potential alternative to or adjunctive treatment for refractory neuropathic pain. However, recent research suggests that ketamine treatment increases the levels of neurotrophins, including brain-derived neurotrophic factor (BDNF) [9,10].
BDNF plays a pivotal role in neural differentiation, growth, survival, and neurotransmission [12]. In the context of adverse events in the central nervous system, there is a concurrent increase in BDNF levels in the cerebrospinal fluid (CSF), indicating the neuroprotective and regenerative potential of BDNF [12,13]. However, during the synaptic plasticity process linked to long-term potentiation (LTP), which is considered a potential contributor to hyperalgesia and allodynia, NMDA receptor signaling and BDNF play crucial roles and mutually interact [12,14].
While many aspects remain to be elucidated, research on TBI-induced neuropathic pain in human subjects is limited by several limitations. In this context, based on our previously validated rat model for mild TBI-induced mechanical allodynia via controlled cortical impact (CCI) [13,15], we aimed to evaluate the impact of intrathecally administered ketamine on BDNF concentration in the CSF (c-BDNF) and the development of allodynia.
Material and Methods
ETHICAL STATEMENT:
All animal protocols received approval from the Institutional Animal Care and Use Committee (PNUH-2019-157). All experiments were performed following the guidelines outlined in the Guide for the Care and Use of Laboratory Animals by the United States National Institutes of Health (NIH Publication No. 85-23).
MATERIALS AND EXPERIMENTAL ANIMALS:
The materials and equipment employed for the experiments in this research are documented in Table 1. Male Sprague-Dawley rats aged 7 weeks and weighing approximately 220 g (Hana Laboratories, Korea) were maintained in a controlled environment at an appropriate temperature. A total of 45 rats were assigned to 3 groups of 15 individuals each, using blocked randomization with the Sealed Envelope system (https://www.sealedenvelope.com/): the sham operation group (Group S), the untreated TBI group (Group T), and the ketamine-treated TBI group (Group K). In subsequent analyses, deceased rats were excluded. Based on a prior study, in which Group S displayed comparable c-BDNF expression and mechanical allodynia to Group C, we only employed the sham-control group for comparison [13].
TBI DELIVERY:
The induction of a mild TBI model was accomplished using the controlled cortical impact (CCI) technique [13,15]. Anesthesia was administered via a combination of oxygen and 5% isoflurane for induction and 2.5% for maintenance. Rats were placed in prone position and their skulls were securely affixed to a stereotactic frame. A craniotomy was performed at the point of 5 mm from the midline of the sagittal suture, utilizing a drill equipped with 5-mm trephine bits. After removing the bone flap, the point of an electromagnetic impactor was placed on the dura. An impact was applied with a 3 m/s velocity, dwell duration of 85 ms, and a depth of 3.5 mm. The scalp closure was accomplished using a surgical skin stapler after all procedures were completed.
KETAMINE TREATMENT:
In Group K, ketamine hydrochloride (Huons Medicare, Busan, Korea) was administered intrathecally immediately after surgery. A 27G disposable scalp vein set was vertically introduced through the atlanto-occipital space, followed by the injection of 10 μL of ketamine (3.41 μg/g) into the cisterna magna. Subsequently, ensuring the unobstructed flow of the cerebrospinal fluid (CSF), the ketamine pre-filled within the scalp vein set was carefully administered.
In Group S, the same procedures, except for the delivery of the CCI, were performed. In Group T, no drug was injected after CCI delivery. Rats of all groups were returned to their home cages for recovery after completion of the procedures. All invasive procedures (surgery, administration of IT ketamine, and CSF sampling) were performed under isoflurane anesthesia (5 vol% for induction, 2.5 vol% for maintenance with oxygen), and aseptic techniques were applied.
MECHANICAL ALLODYNIA ASSESSMENT:
Before the operation, a blinded investigator assessed mechanical allodynia using the von Frey test and the 50% mechanical withdrawal threshold (50% MWT) and repeated these tests on postoperative days (PODs) 1, 7, and 14. Prior to assessment, all rats were habituated to a metal mesh screen for 1 h. With adequate force to cause filament bending for 2 s, a von Frey filament was applied perpendicular to both hind paws from beneath the mesh screen. Eleven von Frey filaments, numbered (bending force in g) from 4.31 [2.04] to 5.16 [14.45], were used and sequentially applied using the up-down method [16], beginning with the filament with the lowest bending force (number 4.31). The evaluations were conducted repeatedly for 6 additional stimuli and recorded. Using Dixon’s approach, the 50% MWT was measured and a von Frey filament of either number 3.538 or 5.272 was applied for stimulation, depending on whether the response was consistently positive or negative [17].
MEASUREMENT OF BDNF IN CSF:
CSF samples were obtained on POD 1, 7, and 14. A 27G scalp vein set connected with a 1-mL syringe was introduced into the atlanto-occipital space under isoflurane anesthesia [13,15]. Specimens <100 μL and manifesting blood tainting were considered inappropriate for analysis and excluded. The included samples were immediately placed in a 4°C refrigerator. After 15 min of centrifugation at 3000 rpm, the collected resultant supernatants were frozen at −80°C.
A BDNF enzyme-linked immunosorbent assay (ELISA) kit (ProteinTech, Boulder, CO, USA) was used to quantify BDNF levels in CSF. We loaded 100 μL of cerebrospinal fluid (CSF) into 96-well plates to allow for the binding of BDNF to the immobilized antibody within the wells over a 150-min period. This was followed by 1-h incubation of the plate with a biotinylated anti-rat BDNF antibody, subsequently proceeding to a buffer wash step. Subsequently, the wells were incubated with horseradish peroxidase-conjugated streptavidin for 45 min. The wells underwent buffer rinsing, followed by a 20-min staining period in darkness with the 3,3′,5,5′-tetramethylbenzidine substrate. Subsequently, a stop solution was applied. The absorbance at 450 nm was evaluated with a spectrophotometer. The test was conducted in duplicate, and the average BDNF level for each sample was considered for the subsequent analysis.
STATISTICAL ANALYSES:
Statistical analyses were performed using SPSS version 25 and MedCalc® software (version 18.11.6; MedCalc Software Ltd., Ostend, Belgium). The results are presented as the median and first and third quartiles (Q1 and Q3). The Kruskal-Wallis test with Conover post hoc multiple comparisons was applied for data analyses. Two-sided
Results
SELECTION PROCESS FOR THE MILD TBI MODEL AND CHANGES IN BODY WEIGHT:
Four of the 45 rats died (1 in Group T on POD 8 and 3 in Group K on the day of the surgery) and thus were excluded from further analyses (Figure 1). The body weights of the rats are listed in Table 2. During the experimental period, we observed no difference in the mean body weight among the 3 groups.
RESULT OF 50% MWT:
On POD 1, Groups T and K exhibited a significantly lower 50% MWT than that of Group S (6.6 [5.7, 8.7] g, 10.0 [6.8, 11.6] g, and 18.7 [11.6, 18.7] g, respectively; P<0.001) (Figure 2).
CSF ANALYSIS AND C-BDNF LEVELS:
The CSF samples included in the analysis are presented in Figure 1. The c-BDNF levels of Group K were significantly higher than Groups S and T on POD 1 (18.9 [16.1, 23.0] pg/ml, 7.3 [6.0, 8.8] pg/ml, and 11.0 [10.6, 12.3] pg/ml, respectively; P=0.006) (Table 3).
Discussion
In this study using a rat model of mild traumatic brain injury, the intrathecal administration of ketamine did not prevent allodynia after TBI. Among the 3 groups, BDNF concentration in CSF on POD 1 was the highest in Group K, and the changes in the 50% MWT were not significantly different between Groups K and T.
Although many studies have demonstrated the positive effects of ketamine on neuropathic pain, most studies have administered ketamine as a combination or adjuvant therapy with opioids and anticonvulsants rather than monotherapy [18–20]. Hence, their results may differ from those of the present study, in which only ketamine was administered. We focused on the effects of intrathecal ketamine administration on the development of neuropathic pain and CSF concentration of BDNF following TBI. To the best of our knowledge, this is the first study to investigate the preventive effects of ketamine against the development of allodynia after TBI.
Ketamine is widely used for the treatment of neuropathic pain. Intravenous ketamine is used as an adjuvant to treat opioid-resistant and intractable chronic neuropathic pain [21]. Ketamine is also administered intrathecally to induce surgical anesthesia. As a noncompetitive antagonist of NMDA receptors, ketamine modulates the ascending nociceptive transmission and descending inhibitory pathways [22]. Blocking the NMDA receptor with ketamine increases the expression of BDNF and tropomyosin receptor kinase B (TrkB) and activates the downstream mammalian target of rapamycin signaling pathway [23–26]. Our findings confirmed that intrathecal injection of ketamine increased c-BDNF expression in vivo. The signaling pathways of these molecules are also involved in the expression of neuropathic pain. Moreover, Group K had a higher incidence of allodynia than Group S and had a rate similar to Group T. We confirmed that intrathecally injected ketamine increased the prevalence of allodynia in a rat model of mild TBI.
Burton et al reported that preemptive intrathecal ketamine injection decreases neuropathic pain behaviors and the anti-allodynic effect of NMDA antagonists on neuropathic pain in rats following nerve injury [27]. They observed that ketamine attenuated the initiation of central sensitization leading to neuropathic pain after peripheral nerve injury, thereby preventing the development of neuropathic pain, which is inconsistent with the present results. Conversely, we investigated TBI-related neuropathic pain following traumatic brain injury. As the underlying mechanism of central neuropathic pain differs from that due to peripheral insults, the effect of ketamine may have differed between the 2 studies. Moreover, while we administered ketamine after the completion of the surgery, Burton et al administered ketamine 15 min before ligating the nerves as a pretreatment. However, preemptively administering ketamine in a TBI model is challenging. Achieving a consistent time of ketamine injection across studies is essential to properly compare these findings.
We assessed mechanical allodynia using the 50% mechanical withdrawal threshold (MWT) [28]. Calculated via Dixon’s formula, significant differences 50% MWT values between experimental groups and controls at specific time points indicated the presence of mechanical allodynia. Furthermore, we identified mechanical allodynia based on reductions in the 50% MWT values before and after the mild TBI.
Through this, we confirmed that high c-BDNF levels were correlated with the development of allodynia. On POD 1, Groups T and K exhibited increased c-BDNF levels and decreased 50% MWT as compared with those of Group S. Subsequently, serial tests performed on POD7 and 14 showed similar c-BDNF levels in Groups T and K, and the 50% MWT was consistently low in both groups. Unlike the significant differences reported earlier between c-BDNF and 50% MWT on POD 1 in Groups T and K, there was no significant difference among the 3 groups in the 50% MWT on POD 7 and 14. Similar to earlier research, our study found a significant correlation between c-BDNF levels and the manifestation of allodynia in a rat model of mild TBI [7,13,15]. However, while a previous study demonstrated a relatively prolonged duration of association between c-BDNF and allodynia, our current study found a comparatively shorter duration of this relationship [15]. Therefore, divergent views may arise regarding the interpretation of high c-BDNF levels as an indicator of persistent allodynia. Although there was no statistically significant variance observed in the 50% MWT of the groups on POD 7 and 14 in this study, conducting an extended study with a larger sample size may clarify whether this trend persists over time or if significant differences develop among the groups.
Our study has several limitations. First, we could not use different ketamine concentrations, which may have helped elucidate the effects of ketamine based on its concentration. Second, administering ketamine at different time points could have led to varied outcomes. As the mechanism of neuropathic pain remains incompletely understood, consistent time frames for ketamine injections after injury must be established to enable comparison across studies. Furthermore, owing to differences in protocols, such as ketamine dosage and infusion periods, further large-scale quantitative studies are warranted to gain a comprehensive mechanistic understanding of the effect of ketamine in development of neuropathic pain. Third, our results were presumed to be derived from a single isomer rather than from a racemic mixture of ketamine. Ketamine contains the enantiomers (R)-ketamine and (S)-ketamine; its neurotoxicity depends on the enantiomeric form. Several cases of subpial vacuolar myelopathy, spinal vessel vasculitis, central chromatolysis, nerve cell shrinkage, microglial upregulation, and gliosis have been reported based on post-mortem histological findings after intrathecal (S)-ketamine injection [29–31]. Furthermore, since each ketamine enantiomer has a different potency and duration of action, its effect as an antidepressant and on BDNF–TrkB signaling and synaptogenesis differs based on the enantiomer [32]. The results of our experiment are similar to those of previous studies [29–31] on the effects of S-ketamine. To prevent this result in advance, an experiment using a single isomer is necessary. As in previous studies, our study focused on the effects of drugs used in vivo in clinical practice, which may have limited our findings. Future research may find differences in the effects of ketamine on the expression of neuropathic pain based on its enantiomers.
Conclusions
In conclusion, our findings suggest that intrathecal ketamine administration did not exert an anti-allodynic effect after mild TBI in a rat model. Nonetheless, it induced an increase in BDNF levels in the CSF, suggesting that c-BDNF could serve as a promising potential indicator of allodynia following mild TBI. Further research on the interactions between ketamine and neurotrophic factors at each stage of neuropathic pain development are needed.
Figures
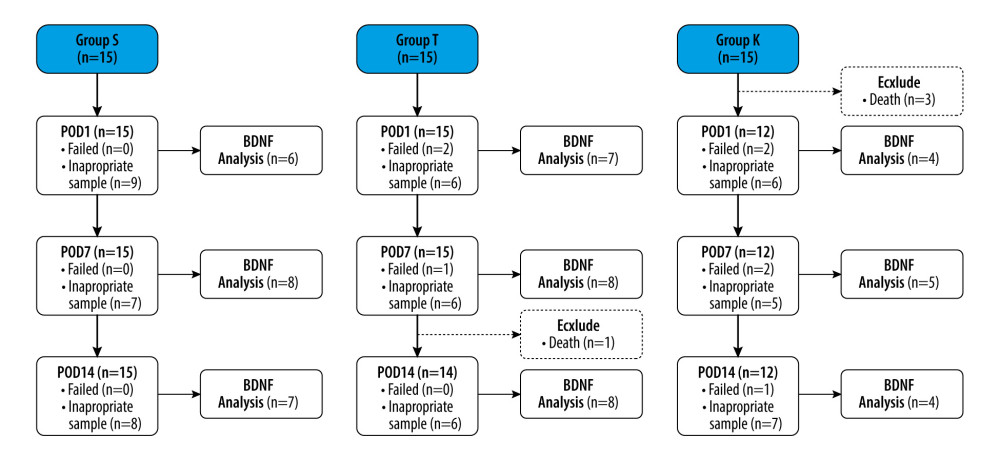
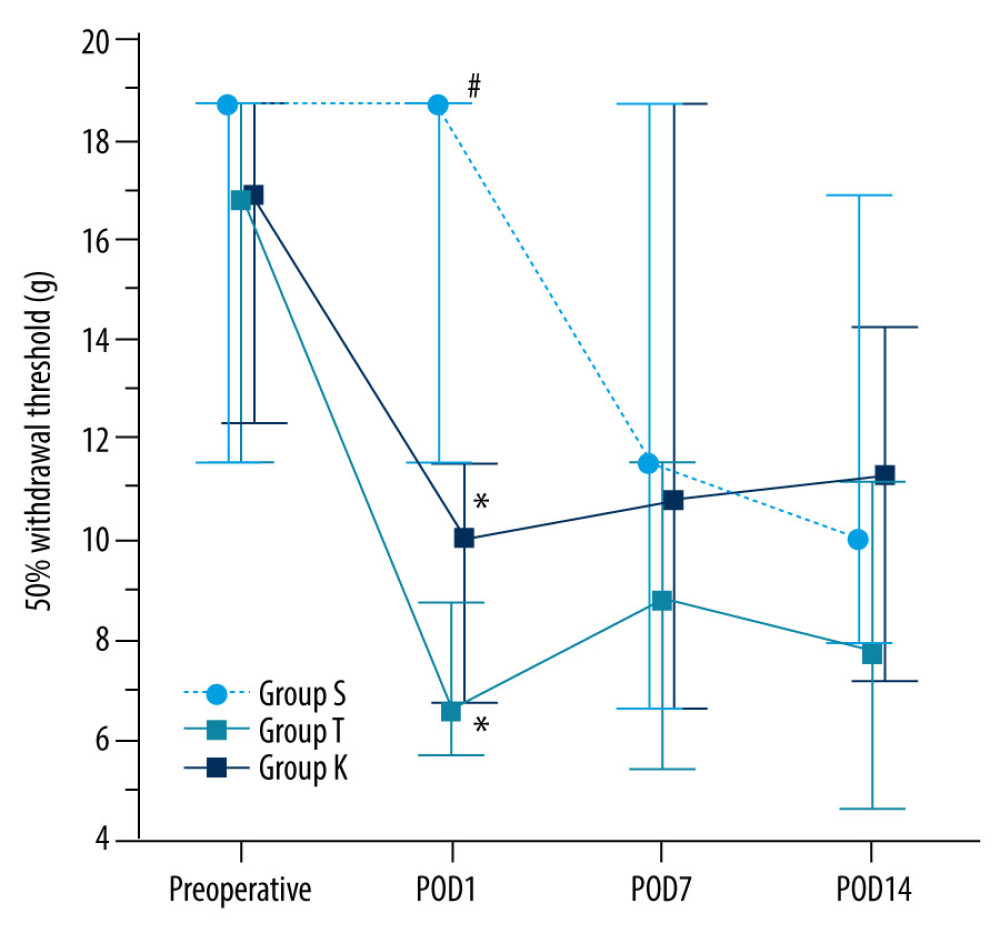
References
1. Hyder AA, Wunderlich CA, Puvanachandra P, The impact of traumatic brain injuries: A global perspective: NeuroRehabilitation, 2007; 22(5); 341-53
2. Harrison-Felix CL, Whiteneck GG, Jha A, Mortality over four decades after traumatic brain injury rehabilitation: A retrospective cohort study: Arch Phys Med Rehabil Sep, 2009; 90(9); 1506-13
3. Widerström-Noga E, Govind V, Adcock JP, Subacute pain after traumatic brain injury is associated with lower insular n-acetylaspartate concentrations: J Neurotrauma, 2016; 33(14); 1380-89
4. Irvine KA, Clark JD, Chronic pain after traumatic brain injury: Pathophysiology and pain mechanisms: Pain Med, 2018; 19(7); 1315-33
5. Jensen TS, Finnerup NB, Allodynia and hyperalgesia in neuropathic pain: Clinical manifestations and mechanisms: Lancet Neurol Sep, 2014; 13(9); 924-35
6. He Y, Kim PY, Allodynia: StatPearls, 2023 Available from: http://www.ncbi.nlm.nih.gov/books/NBK537129
7. Feliciano DP, Sahbaie P, Shi X, Nociceptive sensitization and BDNF up-regulation in a rat model of traumatic brain injury: Neurosci Lett, 2014; 583; 55-59
8. Ikeda K, Hazama K, Itano Y, Development of a novel analgesic for neuropathic pain targeting brain-derived neurotrophic factor: Biochem Biophys Res Commun, 2020; 531(3); 390-95
9. Velzen MV, Dahan JDC, van Dorp ELA, Efficacy of ketamine in relieving neuropathic pain: A systematic review and meta-analysis of animal studies: Pain, 2021; 162(9); 2320-30
10. Guimarães Pereira JE, Ferreira Gomes Pereira L, Mercante Linhares R, Efficacy and safety of ketamine in the treatment of neuropathic pain: A systematic review and meta-analysis of randomized controlled trials: J Pain Res, 2022; 15; 1011-37
11. Rosenbaum SB, Gupta V, Patel P, Ketamine: StatPearls, 2023 Avaliable from: http://www.ncbi.nlm.nih.gov/books/NBK470357
12. Bathina S, Das UN, Brain-derived neurotrophic factor and its clinical implications: Arch Med Sci, 2015; 11(6); 1164-78
13. Do W, Baik J, Jeon S, Increased brain-derived neurotrophic factor levels in cerebrospinal fluid during the acute phase in TBI-induced mechanical allodynia in the rat model: J Pain Res, 2022; 15; 229-39
14. Zhuo M, Long-term potentiation in the anterior cingulate cortex and chronic pain: Philos Trans R Soc Lond B Biol Sci, 2014; 369(1633); 20130146
15. Jeon S, Baik J, Kim J, Intrathecal dexmedetomidine attenuates mechanical allodynia through the downregulation of brain-derived neurotrophic factor in a mild traumatic brain injury rat model: Korean J Anesthesiol, 2023; 76(1); 56-66
16. Chaplan SR, Bach FW, Pogrel JW, Quantitative assessment of tactile allodynia in the rat paw: J Neurosci Methods, 1994; 53(1); 55-63
17. Dixon WJ, Efficient analysis of experimental observations: Annu Rev Pharmacol Toxicol, 1980; 20; 441-62
18. Kim K, Mishina M, Kokubo R, Ketamine for acute neuropathic pain in patients with spinal cord injury: J Clin Neurosci, 2013; 20(6); 804-7
19. Benrath J, Scharbert G, Gustorff B, Long-term intrathecal S(+)-ketamine in a patient with cancer-related neuropathic pain: Br J Anaesth Aug, 2005; 95(2); 247-49
20. Balanaser M, Carley M, Baron R, Combination pharmacotherapy for the treatment of neuropathic pain in adults: Systematic review and meta-analysis: Pain, 2023; 164(2); 230-51
21. Bell RF, Kalso EA, Ketamine for pain management: Pain Rep, 2018; 3(5); e674
22. Maher DP, Chen L, Mao J, Intravenous ketamine infusions for neuropathic pain management: A promising therapy in need of optimization: Anesth Analg, 2017; 124(2); 661-74
23. Singh I, Morgan C, Curran V, Ketamine treatment for depression: Opportunities for clinical innovation and ethical foresight: Lancet Psychiatry, 2017; 4(5); 419-26
24. Peltoniemi MA, Hagelberg NM, Olkkola KT, Ketamine: A review of clinical pharmacokinetics and pharmacodynamics in anesthesia and pain therapy: Clin Pharmacokinet, 2016; 55(9); 1059-77
25. Zanos P, Thompson SM, Duman RS, Convergent mechanisms underlying rapid antidepressant action: CNS Drugs, 2018; 32(3); 197-227
26. Björkholm C, Monteggia LM, BDNF – a key transducer of antidepressant effects: Neuropharmacology, 2016; 102; 72-79
27. Burton AW, Lee DH, Saab C, Preemptive intrathecal ketamine injection produces a long-lasting decrease in neuropathic pain behaviors in a rat model: Reg Anesth Pain Med, 1999; 24(3); 208-13
28. Osier N, Dixon CE, The controlled cortical impact model of experimental brain trauma: Overview, research applications, and protocol: Methods Mol Biol, 2016; 1462; 177-92
29. Vranken JH, Troost D, Wegener JT, Neuropathological findings after continuous intrathecal administration of S(+)-ketamine for the management of neuropathic cancer pain: Pain, 2005; 117(1–2); 231-35
30. Karpinski N, Dunn J, Hansen L, Subpial vacuolar myelopathy after intrathecal ketamine: Report of a case: Pain, 1997; 73(1); 103-5
31. Stotz M, Oehen HP, Gerber H, Histological findings after long-term infusion of intrathecal ketamine for chronic pain: A case report: J Pain Symptom Manage, 1999; 18(3); 223-28
32. Muller J, Pentyala S, Dilger J, Ketamine enantiomers in the rapid and sustained antidepressant effects: Ther Adv Psychopharmacol, 2016; 6(3); 185-92
Figures
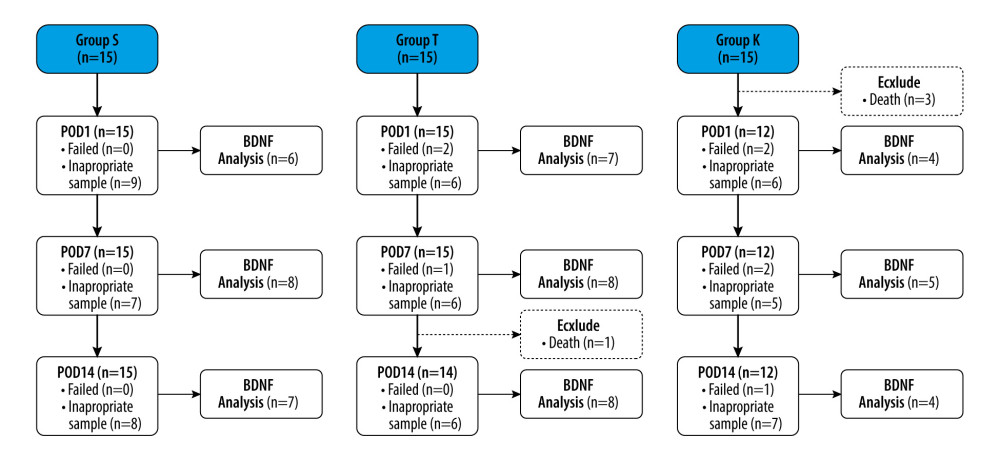
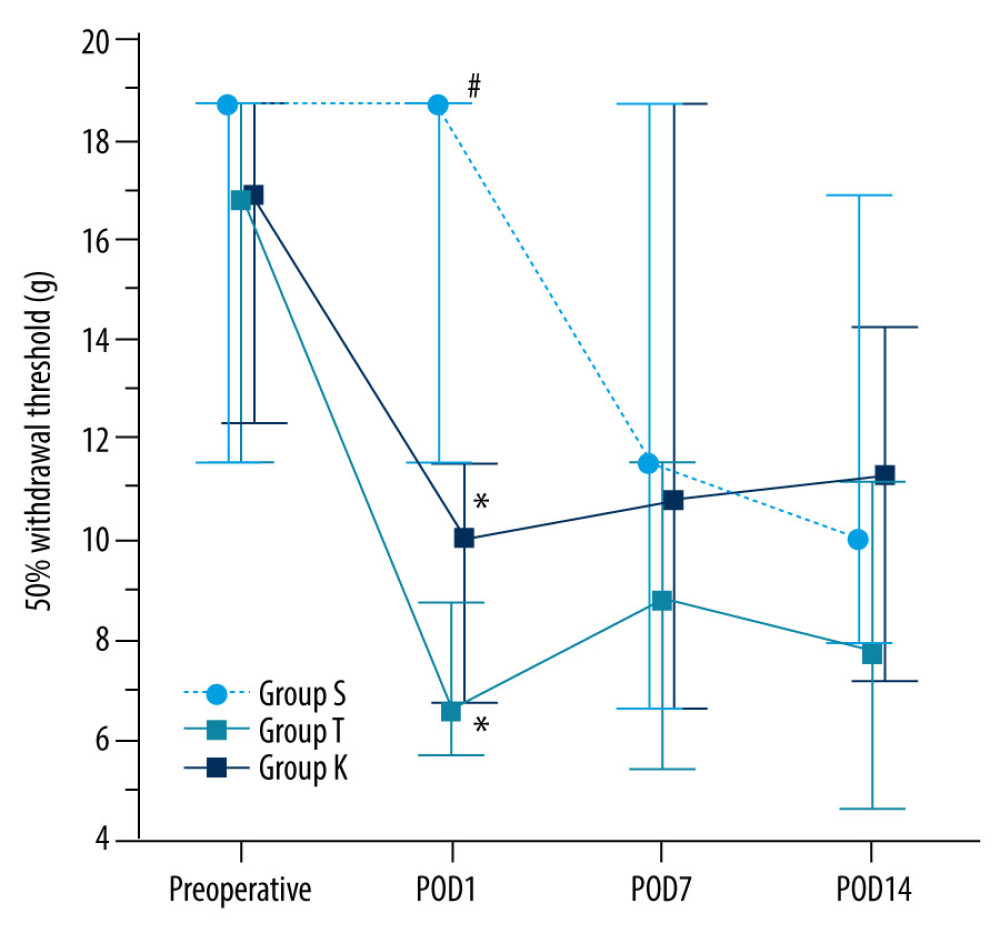
Tables
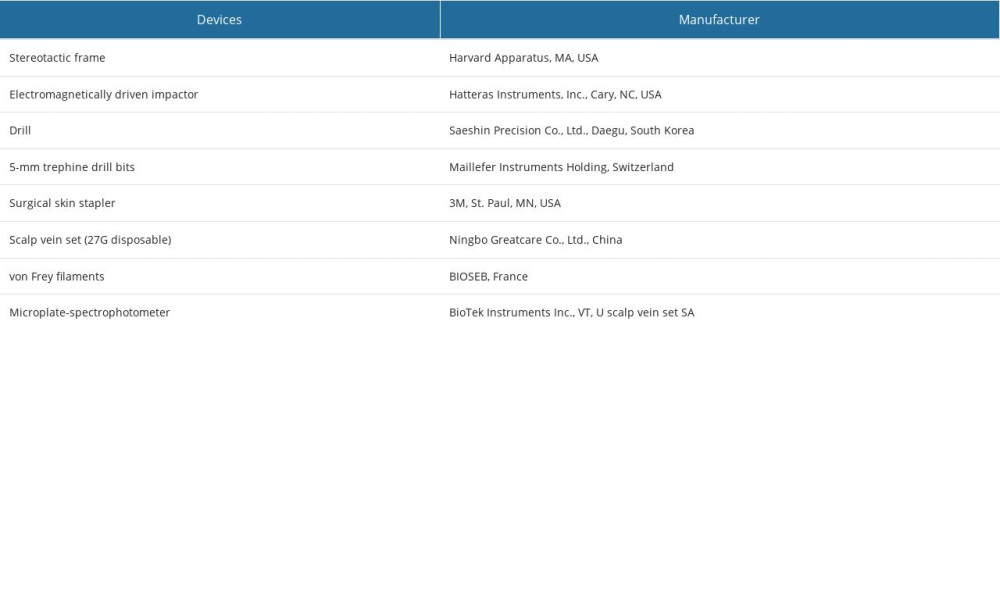
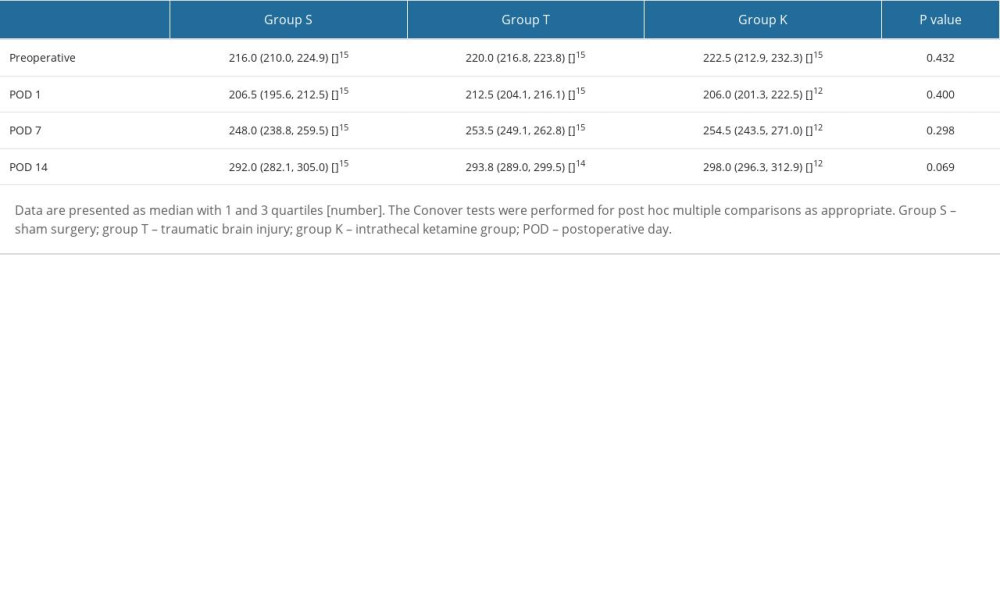
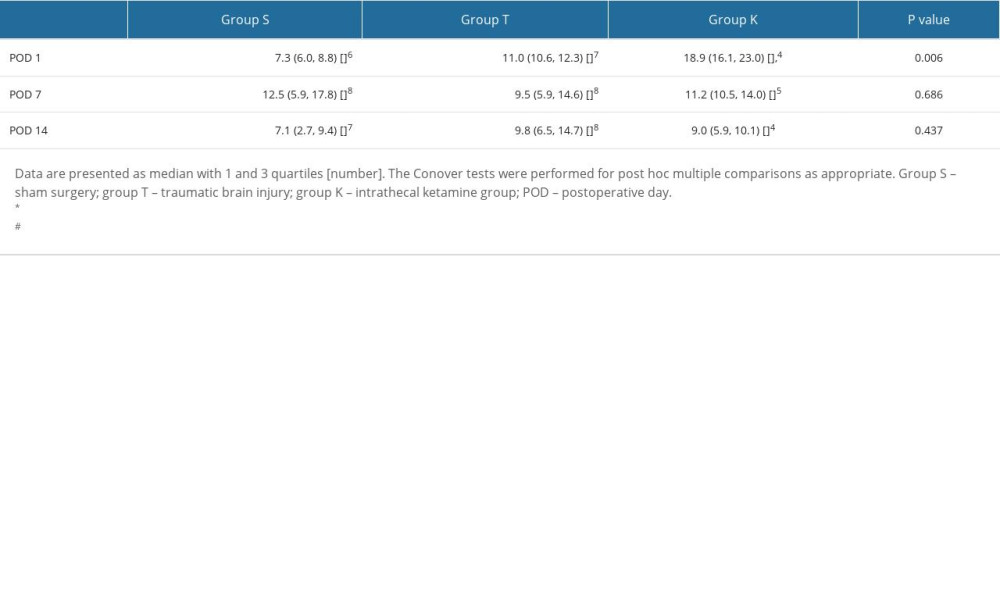
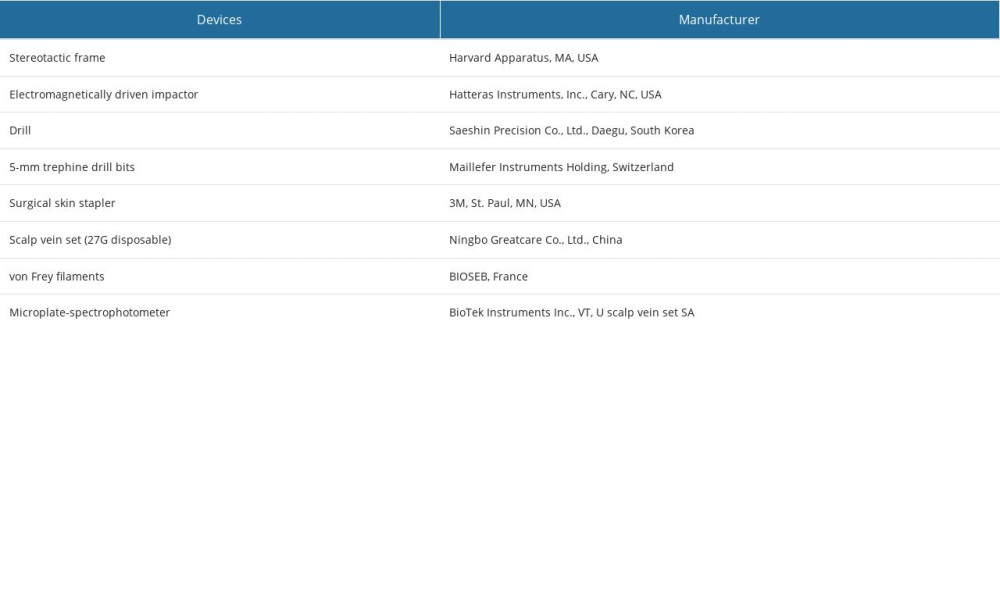
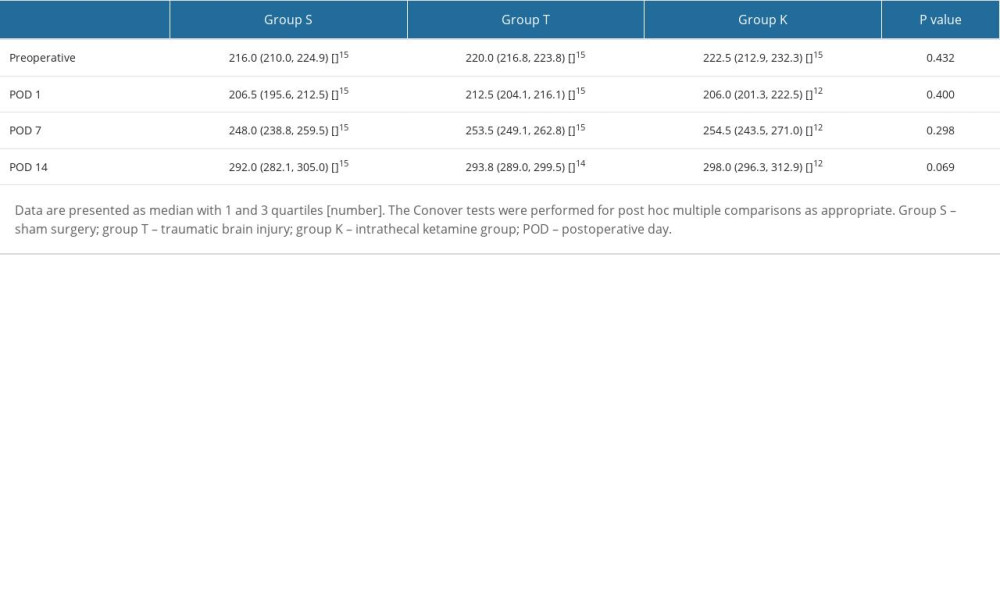
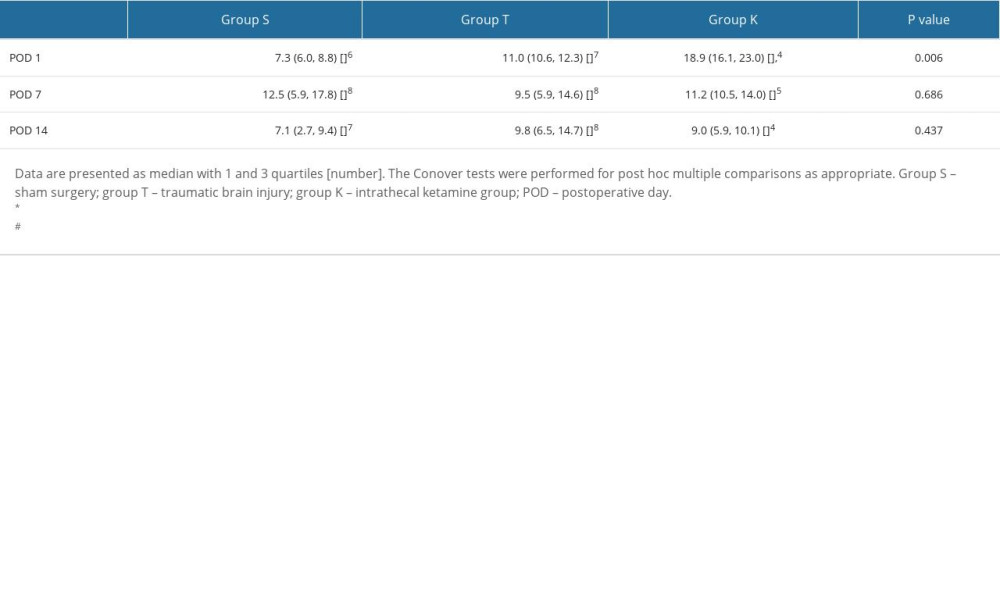
In Press
08 Mar 2024 : Animal Research
Modification of Experimental Model of Necrotizing Enterocolitis (NEC) in Rat Pups by Single Exposure to Hyp...Med Sci Monit In Press; DOI: 10.12659/MSM.943443
18 Apr 2024 : Clinical Research
Comparative Analysis of Open and Closed Sphincterotomy for the Treatment of Chronic Anal Fissure: Safety an...Med Sci Monit In Press; DOI: 10.12659/MSM.944127
08 Mar 2024 : Laboratory Research
Evaluation of Retentive Strength of 50 Endodontically-Treated Single-Rooted Mandibular Second Premolars Res...Med Sci Monit In Press; DOI: 10.12659/MSM.944110
11 Mar 2024 : Clinical Research
Comparison of Effects of Sugammadex and Neostigmine on Postoperative Neuromuscular Blockade Recovery in Pat...Med Sci Monit In Press; DOI: 10.12659/MSM.942773
Most Viewed Current Articles
17 Jan 2024 : Review article
Vaccination Guidelines for Pregnant Women: Addressing COVID-19 and the Omicron VariantDOI :10.12659/MSM.942799
Med Sci Monit 2024; 30:e942799
14 Dec 2022 : Clinical Research
Prevalence and Variability of Allergen-Specific Immunoglobulin E in Patients with Elevated Tryptase LevelsDOI :10.12659/MSM.937990
Med Sci Monit 2022; 28:e937990
16 May 2023 : Clinical Research
Electrophysiological Testing for an Auditory Processing Disorder and Reading Performance in 54 School Stude...DOI :10.12659/MSM.940387
Med Sci Monit 2023; 29:e940387
01 Jan 2022 : Editorial
Editorial: Current Status of Oral Antiviral Drug Treatments for SARS-CoV-2 Infection in Non-Hospitalized Pa...DOI :10.12659/MSM.935952
Med Sci Monit 2022; 28:e935952