20 September 2023: Clinical Research
Early Echocardiography and ECG Changes Following Radiotherapy in Patients with Stage II–III HER2-Positive Breast Cancer Treated with Anthracycline-Based Chemotherapy with or without Trastuzumab-Based Therapy
Radu Valeriu Toma- A Study design/planning
- B Data collection/entry
- C Data analysis/statistics
- G Funds collection
- A Study design/planning
- D Data interpretation
- *Corresponding author: [email protected]
- *Corresponding author: [email protected]
- C Data analysis/statistics
- D Data interpretation
- E Preparation of manuscript
- F Literature analysis/search
- A Study design/planning
- B Data collection/entry
- C Data analysis/statistics
- D Data interpretation
- E Preparation of manuscript
- F Literature analysis/search
DOI: 10.12659/MSM.941754
Med Sci Monit 2023; 29:e941754
Abstract
BACKGROUND: Cardiotoxicity from radiotherapy and anti-cancer therapies have been reported in patients with breast cancer. This study aimed to investigate the early echocardiography and ECG changes following radiotherapy in 68 patients ages 30-78 years with stages II-III HER2-positive breast cancer treated with anthracycline-based chemotherapy with or without trastuzumab-based therapy from 2015 to 2021.
MATERIAL AND METHODS: We analyzed data of 68 breast cancer patients aged 30-78 years, predominantly in AJCC stages II-III (61) and HER2-positive (58), treated and monitored from 2015 to 2021. Cardiac function was assessed using echo- and electrocardiography. We employed univariate logistic models to gauge associations between pre-existing cardiac conditions, treatment modalities, and changes in cardiac function.
RESULTS: A decrease in the left ventricle ejection fraction (EF) by >5% was associated with heart doses >49.3 Gy and with maximum and average doses to the left anterior descending artery (LAD) exceeding 46.9 Gy and 32.7 Gy, respectively. An EF drop of ≥10% was correlated with anti-HER2 therapy, pre-existing ECG changes, and the onset of conditions in the left ventricle, major vessels, and valves. Conditions were exacerbated in patients with prior echocardiographic abnormalities, while some emerged concurrent with the EF decline.
CONCLUSIONS: This research emphasizes the importance of personalized heart monitoring and care for breast cancer patients undergoing multimodal therapies. Significant and potentially irreversible EF declines can result from radiation and anti-HER2 treatments.
Keywords: Breast Neoplasms, chemoradiotherapy, Anthracyclines, Echocardiography, Female, Humans, Electrocardiography, Receptor, erbB-2, Trastuzumab
Background
Breast cancer is the most prevalent form of cancer among women worldwide [1–3]. New treatment strategies have increased the survival rates of breast cancer, with a current 5-year survival of 89% for all stages combined [1]. According to Eurostat [4], breast cancer was the leading malignancy among Romanian women in 2020, and the second most common malignancy overall, resulting in approximately 12 000 new cases, nearly 4000 deaths, and a 5-year prevalence exceeding 45 000. In 2017, within the European Union (EU), breast cancer claimed the lives of 84 500 female patients. This death toll accounted for 7.3% of all cancer-related deaths in the EU, and breast cancer specifically was responsible for 16.5% of all cancer-related deaths in females [4].
While breast cancer treatment has yielded progressively encouraging outcomes, certain adjuvant therapies, such as radiotherapy, can result in heightened occurrences of cardiovascular adverse events [1,5,6]. This is significant because cardiovascular disease has emerged as the leading cause of non-cancer-related mortality among women diagnosed with breast cancer [7,8]. With the advancements in oncological treatment over the past few decades, patients diagnosed with left breast cancer now experience prolonged overall survival [9]. In Europe, the 5-year survival rate for patients with left breast cancer, who face a higher risk of cardiovascular adverse events, has increased to 88.1% [10].
The frequency of cardiac adverse events from conventional cancer treatments, including chemotherapy with anthracyclines [11,12], hormonotherapy, anti-HER2 therapy, and radiotherapy, is higher than previously estimated, largely due to improved patient survival; for HER2+ breast cancer patients in particular, the significant survival benefits from anti-HER2 agents and chemotherapies introduce an associated risk of cardiotoxicity, highlighting the essential role of monitoring and understanding these cardiac events as patients live longer due to these effective treatments [13–15]. As a result, they emerged as the leading cause of morbidity and mortality among cancer survivors and a new subspecialty has emerged – cardio-oncology [16] – dealing with cardiovascular pathological conditions in multimodally-treated patients, including those with myocardial infarction or heart failure, which often manifest after an extended follow-up period [1,10].
Radiotherapy (RT) is a therapeutic modality employed in multimodal treatment for breast cancer, aiming to enhance local tumor control and reduce mortality risk [17]. However, chest radiotherapy has been associated with an increased incidence of heart conditions among cancer survivors [18]. In recent decades, significant progress has been made in the field of radiotherapy, particularly in improving the distribution of radiation while minimizing its impact on the heart [19]. There has been a rapid evolution from 2D irradiation to high-performance approaches such as 3D conformational irradiation or intensity-modulated radiotherapy (IMRT), image-guided radiotherapy (IGRT), and volumetric modulated arc therapy (VMAT) [20].
Furthermore, the use of helical tomotherapy, respiratory gating, ventral decubitus positioning, and partial breast irradiation enhances the precision of treatment [21–23]. However, irradiation of the heart is inevitable in all radiation therapies, and the entire extent and mechanisms of irradiation’s effects on the heart is unknown [24,25]. Consequently, the incidence of radiation-induced heart conditions is anticipated to rise among cancer survivors who underwent older radiotherapy techniques, even though modern radiotherapy methods have reduced their occurrence and severity [26].
In this paper, we sought to identify clinical and treatment-related factors associated with early changes in electro- and echocardiographic measurements following therapy. The purpose of this investigation was to provide guidance for monitoring groups at risk of post-treatment cardiotoxicity. In terms of treatment-related variables, we aimed to determine the specific threshold values for radiation therapy (RT) and chemotherapy (CHT) doses, which are often underreported in the existing literature. These values are critical in understanding the onset of early subclinical cardiac effects and their association with the progression of pre-existing cardiac comorbidities. Additionally, we aimed to estimate the time intervals between the completion of radiotherapy, initiation of chemotherapy, anti-HER2 therapy, and the occurrence of these early events, as well as changes in the diagnostic grading of pre-existing cardiac pathologies. Furthermore, we sought to identify areas for improvement in the sensitivity of instruments and the protocol’s effectiveness in monitoring the cardiac electrical and mechanical functions of breast cancer patients after treatment, particularly those with pre-existing cardiac comorbidities or risk factors at the time of diagnosis.
Therefore, this study aimed to investigate the early echocardiography and ECG changes following radiotherapy in 68 patients aged 30–78 years with stages II–III HER2-positive breast cancer treated with anthracycline-based chemotherapy with or without trastuzumab-based therapy between 2015 and 2021.
Material and Methods
STUDY COHORT:
The authors conducted a population-based case-control analysis on cardiovascular (CV) events in Romanian patients who underwent external-beam radiation for breast cancer treatment at the Oncological Institute in Bucharest between 2015 and 2021. This study was conducted in accordance with the Declaration of Helsinki and Good Clinical Practice guidelines. Ethics approval for this retrospective case-control analysis was granted by the Ethics Committee of the Oncological Institute in Bucharest (Approval Number: EC-3637-22.03.2023). All patients who were included in this study had provided written informed consent for their medical data to be used for research purposes, with the assurance that all data would be anonymized to protect their identity.
Patients in this study were diagnosed with stage II–III breast cancer based on tumor size, extent of lymph node involvement, and absence of distant metastasis, as defined by the American Joint Committee on Cancer (AJCC) staging criteria [27]. The diagnosis was supplemented by comprehensive patient medical histories, tumor characteristics, and treatment details, as found in the records of the Oncology Department. Following the diagnosis, patients were subjected to a multimodal treatment strategy, as determined by a multidisciplinary healthcare team in accordance with the National Comprehensive Cancer Network (NCCN) guidelines.
The identification of cardiovascular events (CVEs) was based on findings from electrocardiographic and echocardiographic examinations. The echocardiographic and electrocardiographic events were categorized and coded using the American College of Cardiology/American Heart Association ACC/AHA guidelines [28,29] and the Minnesota Code Manual of Electrocardiographic Findings [30] (refer to Supplementary Tables 1 and 2 from the Supplementary Materials, respectively).
Each patient was subjected to a standard 12-lead ECG in a quiet, dimly lit room, with the patient lying in a supine position. The skin was first prepped with an alcohol pad to ensure optimal electrode adherence and to reduce potential artifacts. Electrodes were placed on the patient’s limbs and chest, following the standard positioning according to the American College of Cardiology/American Heart Association guidelines (ACC/AHA) [29]. After securing all leads, a resting ECG was recorded for a duration of 10 min. The resulting electrocardiograms were digitally stored and analyzed by a certified cardiologist. Abnormal findings, such as arrhythmias, ST-segment deviations, and Q-wave abnormalities, were categorized and coded in accordance with the Minnesota Code Manual of Electrocardiographic Findings [30].
Patients underwent standard 2D transthoracic echocardiography (TTE) capturing key views like the parasternal long-axis and apical four-chamber. Emphasis was on assessing left ventricular ejection fraction (LVEF) and heart valves functionality [29].
Additionally, we examined patient records from the Oncology Department to gather information on each patient’s medical history prior to their breast cancer diagnosis, tumor characteristics, and treatment.
ELIGIBILITY CRITERIA:
Patients were eligible for inclusion if they were diagnosed with breast cancer and were above 18 years of age, had a sinus heart rhythm during the baseline cardiologic examination, or received either anthracyclines (AC) or Trastuzumab (TRZ), or both, if radiotherapy-related data were available. Patients were excluded if they met any of the following criteria: lack of histological evidence of breast cancer, presence of bilateral or metastatic disease at the time of diagnosis, history of other malignancies, previous thoracic radiation, prior exposure to anthracyclines or other neoadjuvant CHT, life expectancy shorter than 12 months, participation in an oncology clinical trial, history of myocardial infarction or heart failure, pregnancy and/or lactation, or left ventricular ejection fraction (EF) <50%, indicating impaired cardiac function prior to RT.
MULTIMODAL TREATMENT STRATEGY:
The treatment protocol was determined by a multidisciplinary healthcare team in accordance with NCCN guidelines. For T1 or T2 staged tumors without lymph node involvement, patients have the option of receiving either lumpectomy followed by whole-breast radiation therapy with a boost to the tumor bed or mastectomy without adjuvant RT. In cases of N0 staged lymph nodes, RT is not administered to local lymph node groups, unless fewer than 10 lymph nodes were removed during surgery. For T3 and T4 staged tumors and/or N1 staged lymph nodes, patients undergo local treatment (mastectomy or lumpectomy), followed by CHT (before or after surgery) and adjuvant RT to the chest wall and lymph nodes.
Breast-conserving surgery is the standard approach for tumors measuring 2 cm or less, except for aggressive phenotypes such as triple-negative breast cancer. If the tumor size exceeds 2 cm and breast conservation is intended, systemic therapy can be attempted as a primary option. If the treatment response is satisfactory, breast-conserving surgery is performed; otherwise, mastectomy is pursued. If breast conservation is not desired or feasible, mastectomy with or without reconstruction is the standard approach.
When considering neoadjuvant systemic treatment, it is generally not recommended for T1N0 or T2N0 disease. However, the histological type and immunohistochemical profile can impact the oncologist’s decision to administer chemotherapy [31]. In specific cases, neoadjuvant systemic therapy may be advantageous for HER2-positive breast cancer patients or those with triple-negative very early breast cancer [32]. For tumors staged as T3 or T4 and/or lymph nodes staged as N1, a commonly employed treatment regimen involves 4 cycles of epirubicin and cyclophosphamide, followed by taxanes (docetaxel or paclitaxel) [31]. If this regimen is not administered prior to surgery, these patients can still benefit from adjuvant chemotherapy using the same treatment protocol.
Adjuvant endocrine therapy is administered to patients with hormone receptor-positive disease, premenopausal patients undergoing ovarian function suppression (OFS), and either tamoxifen or an aromatase inhibitor. Postmenopausal women with hormone receptor-positive cancer are advised to take tamoxifen or aromatase inhibitors for a minimum of 5 years. In the case of HER2-positive disease, adjuvant anti-HER2 therapy is recommended for a duration of 1 year [33].
RADIOTHERAPY SIMULATION, CONTOURING, AND PLANNING:
The reconstruction of RT fields on a computed tomography (CT) scan necessitated virtual simulation and CT planning. For treatment planning, data acquisition involved using a CT simulator with contrast, immobilization, and positioning. The patient was placed in the supine position, lying on their back, using a “QUEST” metal frame inclined at 15–20°. The upper limbs were supported symmetrically, in abduction, at approximately 120°. CT images at a 3–5 mm slice thickness were utilized for this purpose.
The vascular structures at risk, namely the left coronary artery (LCA), the right coronary artery (RCA), the left anterior descending artery (LAD), and the anterior circumflex artery (ACX), were obtained through manual segmentation of planning CT images. These structures are depicted in a three-dimensional reconstruction in Figure 1A. Figure 1B illustrates the anatomical relationships between these arteries and the walls of the heart’s largest cavity, the LV, while Figure 1C shows the relationships of the coronary vasculature with the ascending aorta. Lastly, Figure 1D displays the anatomical relationships of the coronary vasculature with the 2 major vascular tracts, namely the ascending aorta (AA) and the pulmonary trunk (PT), as well as their connections with the walls of the 2 ventricles.
Three-dimensional (3D) reconstruction is a valuable technique in radiotherapy. In the process, cardiac structures are first mapped or contoured from imaging studies, such as CT scans. These contours are then translated into a 3D model using dedicated software. This visual aid allows professionals to pinpoint the radiation’s spatial distribution within the heart. Superimposing the radiation dose on the 3D cardiac model provides a clear understanding of the dose received by each heart structure. By integrating these data, correlations can be made between radiation exposure and potential cardiac complications, enhancing the precision and safety of treatments.
Eclipse® (Varian Medical Systems, Inc.) served as the contouring tool for patients undergoing 3D conformal-RT, while the Monaco® program (Elekta) was utilized for patients treated with VMAT. The contouring process was performed by a radiotherapist, with occasional assistance from a radiologist, following the guidelines set by the Radiation Therapy Oncology Group (RTOG) [34,35] and the recommendations proposed by Feng et al [36]. This approach significantly enhanced the accuracy of delineating cardiac and coronary structures. Supplementary Figure 1 in Supplementary Materials displays the cardiac structures visible in conventional medical imaging, including the left atrium, right atrium, ascending aorta, right coronary artery, right ventricle, left anterior descending artery, left ventricle, and anterior circumflex artery. Among these structures, only LV, RCA, LAD, and ACX are notably susceptible to radiation treatment for left breast cancer.
PRIMARY VARIABLES: In this study, we utilized both primary and secondary variables. The primary variables’ values were obtained from medical records, while the secondary variables were defined based on the primary variables, as illustrated below. All the variables included in this study are listed in Supplementary Tables 3–10 from the Supplementary Materials. We employed 8 categories of data (refer to Table 1), and we represent the index of these categories as ℓ. Within each class, the numerical variables are denoted as xi(ℓ), the binary ordinal variables as yi(ℓ), and the multinomial variables as zk(ℓ).
Throughout this paper, we will utilize the terms “time period” or “time interval” to refer to the duration between the occurrence of an event and one of the following dates: the completion of chemo- or radiotherapy, or the commencement of anti-HER2 therapy, for each patient.
“Event” was defined as an EF loss of over 5% in comparison to the previous echocardiographic examination, the emergence of a new condition, or the deterioration of a pre-existing condition that can be diagnosed through echocardiography and classified according to the aforementioned guidelines [28,30]. The ACC/AHA Guidelines for the Clinical Application of Echocardiography [28] typically define 7 abnormality classes. However, due to the relatively frequent diagnosis of “diastolic dysfunction – delayed relaxation,” we have included it as a distinct class. Nevertheless, in the ACC/AHA Guidelines for the Clinical Application of Echocardiography [28], it is categorized as a subset of “ventricular or diastolic dysfunctions” within the “cardiomyopathies and left ventricular conditions” class.
Cardiac monitoring for our group of patients involved clinical assessments conducted by a cardiologist, electrocardiography, and measurements of EF by echocardiography. These evaluations were performed at the beginning of the study and then at months 3, 6, 12, 18, and 24. Additional assessments were conducted as necessary, depending on the patients’ concerns, in accordance with the most recent cardio-oncology guideline [37]].
All statistical analyses were performed using IBM® SPSS® version 26. The following descriptive statistics – mean value (plus standard error), median, variation, standard deviation, extremes, amplitude, and distribution deviation, as well as the 25%, 33%, 50%, 67%, and 75% percentile values – were calculated for all primary variables (refer to Supplementary Materials Supplementary Tables 2–8). Each variable was checked to see if it had a Gaussian distribution by applying the Kolmogorov-Smirnov test (KS test). Pearson correlation coefficients were determined for normal distribution quantitative variables and Spearman correlation coefficients for variables that do not have a normal distribution.
SECONDARY VARIABLES: Let us now suppose that the variable zk(ℓ) has ζ values (ζ is a positive integer and ζ > 2), then there are n=ζ−1 variables z˜k,n(ℓ) are obtained by regrouping these values. The following example will illustrate how the values of z˜k,n(ℓ) are obtained by regrouping the values of zk(ℓ) variables. Let us suppose that zk(ℓ) is the pre-treatment (ℓ=3) tumor N-staging with ζ=4 values, {N0 or Nχ, N1, N2, N3} then there are n=4 variables z˜k,n(ℓ) defined as follows z˜k,n(ℓ)=0 if zk(ℓ)∈{N0 or Nχ} and z˜k,n(ℓ)=1 if z˜k(ℓ)∈{N1,N2,N3}, z˜k,2(ℓ)=0 if zk(ℓ)∈{N0 or Nχ,N1} and z˜k,2(ℓ)=1 if zk(ℓ)∈{N2, N3} and, finally z˜k,3(ℓ)=0 if zk(ℓ)∈{N0 or Nχ,N1,N2} and z˜k,3(ℓ)=1 if zk(ℓ)∈{N3}. See Supplementary Table 3 from the Supplementary Materials for the obtained grouping models according to this classification method.
The variables xi(ℓ) and zk(ℓ) were transformed into the binary ordinal variables x˜i,α(ℓ) and z˜k,n(ℓ) as follows. We denote by xi,α(ℓ)– the αth-percentile of the variable xi(ℓ) (α∈{25,33,50,67,75}. When α=50, xi,50(ℓ) is the median (miℓ) corresponding to the variable xi(ℓ). If xi(ℓ)<xi,α(ℓ), then x˜i,α(ℓ)=0, while if xi(ℓ)≥xi,α(ℓ), then x˜i,α(ℓ)=1. Consider, for example, that the variable xi(ℓ) is the weight of the patient at the diagnosis, which is a variable of class ℓ=1 whose 50th percentile xi,50(ℓ) is 70 kg. The secondary variable defined based on this value is xi,50(ℓ) and its values are 0 if the patient’s weight is <70 kg and 1 if the patient’s weight is ≥70 kg. See Supplementary Tables 4–10 from the Supplementary Materials for the values of the percentiles corresponding to all reported variables.
RISK ANALYSIS AND LOGISTIC REGRESSION:
Finally, we estimated through risk calculations – the odds ratio (OR) and risk ratio (RR) – corresponding to the associations between classes of categorical binary variables in the following categories: clinical data and comorbidities, hormonal receptor constellation, treatment, pre-existing echocardiographic and electrocardiographic conditions, and radiotherapy on the one hand, and the outcome categorical binary variables describing refined echocardiographic and electrocardiographic changes on the other hand. We will explain the principle of OR and RR calculation in section 3.3. The statistical significance (
Results
PATIENTS:
This study included 68 patients (refer to Table 2) aged 30–78 years, with a mean age of 55.25 years and a standard deviation of 13.29 years. Most of the patients (71.4%) were in menopause at the time of diagnosis, and nearly half of them (48.6%) were overweight or obese, with an average body mass index (BMI) of 27.8 kg/m2. At the time of diagnosis, over half of the patients (57.8%) with available clinical data had either cardiovascular diseases or diabetes mellitus (DM)/metabolic syndrome (MS) with a non-negligible cardiovascular risk, or both. Moreover, more than half (52.8%) of the patients with cardiovascular diseases exhibited severe forms of these conditions. A small proportion of the patients had inflammatory diseases, with chronic infections being the most common. Additional descriptive statistics for age, weight, BMI, and body surface area (BSA) can be found in Supplementary Table 4 of the Supplementary Materials.
There are associations of statistical significance between certain variables presented in Table 2, as illustrated in Supplementary Figure 2 from the Supplementary Materials. In our sample, female patients aged over 53 years were in a menopausal state. This physiological condition renders patients more susceptible to heart disease, and in our group, it is associated with a weight exceeding 79 kg, a BMI of approximately 27 kg/m2, and a BSA greater than 1.92 m2. The presence of pre-existing comorbidities, particularly cardiovascular diseases, is associated with the same BSA values, but with ages exceeding 48 years and a higher BMI (over 31.65 kg/m2), while adhering to a lower weight threshold (60 kg).
Most patients (70.6%) had cancer located in the left breast (refer to Supplementary Table 4 in Supplementary Materials). A significant portion of patients (75.6%) were staged as T1–T2, nearly half (49.2%) were staged as Nx or N0, and the remaining patients were staged as N1–N3, with most being staged as N1 (32.2%). Overall, except for a small percentage (8.2% of patients in stages IA–IB), most patients had an AJCC staging of II–III. After post-therapeutic restaging, the number of patients classified as stages IA and IB tripled (24.6% post-therapeutic versus 8.2% pre-therapeutic), while 9.8% were classified as stage 0.
Most patients (85.3%) were HER2-positive: 51.5% were double-plus, and 33.8% were triple-plus (refer to Table 3). Furthermore, some of these patients also presented receptors for progesterone (PR) (48.5%) and estrogen (ER) (63.2%). Table 3 illustrates the concurrent presence of hormone receptors within the study group. For detailed statistics on ER and PR frequencies, please refer to Supplementary Table 7 from the Supplementary Materials.
MULTIMODAL THERAPY MODALITIES:
Most patients (88.2%) underwent surgery, while the remaining patients (11.8%) followed therapeutic modalities determined after biopsy (refer to Table 4). Mastectomy was the preferred surgical technique in 77.7% of cases. A similar proportion of patients with amplified HER2 (78.0%) received anti-HER2 treatment, specifically TRZ (92.5%). Radiotherapy was administered to a larger proportion of patients (89.7%), whereas a slightly smaller group (83.8%) received chemotherapy with ACs. Only 86.0% of these patients (49 individuals) were able to complete the 4 cycles of chemotherapy. Refer to Supplementary Table 6 in the Supplementary Materials for descriptive statistics on the prescribed doses for the 4 cycles of anthracycline chemotherapy. The percentile values serve as threshold values for calculating the impact on changes in cardiological findings as described by echocardiography and ECG.
Most patients (89.7%) followed a course of RT, the therapeutic intention in most cases (95.1%) being adjuvant. Among patients treated with 2D conformal irradiation (45.9%), there are no dosimetric variables available for the heart’s vascular and muscular structures. In contrast, the descriptive statistics for these variables can be found in Supplementary Table 8 from the Supplementary Materials for patients who received 3D conformal irradiation (44.3%) and VMAT (9.8%).
In Supplementary Figures 3 and 4 in Supplementary Materials, we present typical cases of RT plans for patients undergoing left and right breast mastectomy. These cases demonstrate that patients with left breast cancer are more likely to experience higher irradiation of cardiac structures, particularly in 3D conformational radiotherapy (Supplementary Figure 3A, 3C). The application of RT with the VMAT technique is clearly more beneficial (Supplementary Figure 3B, 3D). In cases of right breast cancer, the risk of cardiac irradiation significantly decreases regardless of the technique employed (Supplementary Figure 4 in Supplementary Materials).
LEFT VENTRICULAR EJECTION FRACTION DROP:
The patients were monitored echocardiographically (see Supplementary Table 8 in Supplementary Materials) over a period that varies over the entire study group from 4 months (120 days) to 12.5 years (4500 days), the mean and median of the follow-up period being 28.6 months and 21 months. The initial drop in EF of more 5% shortly after the completion of radiation therapy (Figure 2) is usually linked to ECG changes in the anterior lead(s) and affects the morphology of the T wave. The occurrence of this type of EF decrease starting from the day after the initiation of anti-HER2 therapy is associated with values exceeding the 75th percentile, which correspond to the mean and maximum doses of approximately 32.70 and 46.95 Gy, respectively, and the V25 isodose of 70.7 ccs at the level of the LAD. Additionally, the mean dose (approximately 49.3 Gy) and V25 (10.65 ccs) at the level of the entire heart are also implicated (refer to Supplementary Table 11 in Supplementary Material for more details on these cut-off calculations, illustrated by an example from Figure 2).
To verify possible causal synergies, we included the above variables in multinomial logistic models predicting a minimum 5% decrease in EF during and after RT administration by regrouping the variables in Figure 2 as follows: T wave changes and ECG changes in previous leads, each of these separately together with RT variables corresponding to LAD and the whole heart, RT variables corresponding to LAD, RT variables corresponding to the whole heart, and different combinations of the last 2 categories of variables. No statistically significant values (P<0.05) were obtained for the odds ratios (OR) in any of these models. Under these conditions for our study group, each of the conditions listed in Figure 2 can be considered as a predictor for the subclinical decrease in EF peri- and post-RT. Thus, in our study group, no synergy could be identified between the global irradiation of the heart and the irradiation of any coronary vessel.
The mechanical function of the heart continues to deteriorate due to a consistent decrease in EF, which is measured relative to the end of CHT. This deterioration is associated with a pathological preconditioning, indicating the presence of pre-existing cardiac damage. Additionally, a subclinical preconditioning is observed before the initiation of multimodal treatment, as evidenced by ECG changes in the posterior leads, especially morphological changes in the T waves (refer to Figure 3).
The post-therapeutic decrease in EF persists, accompanied by an initial decrease of more than 5% detected during the first echocardiographic control after CHT or RT. This decrease is also linked to new ECG changes recorded in the posterior leads. The progression of these conditions is further correlated with multimodal treatment, specifically the implementation of all therapies, including surgery, CHT, RT, and anti-HER2 therapy. Furthermore, it is associated with mean LAD doses exceeding 32.7 Gy and AC doses surpassing 160 mg/m2 during the third cycle.
Systematically exploring all parameter combinations listed in Figure 3, with 2 exceptions, did not yield systematic multinomial logistic models that included 2 or more predictors of post-CHT EF impairment. The estimated parameters of the 2 statistically significant models can be found in Supplementary Table 12 in the Supplementary Materials. In both models, the main predictor of post-CHT EF degradation is the pre-existence of cardiac pathologies (Model 1, OR=21.82, P=0.021; Model 2, OR=21.34, P=0.002) at the time of diagnosis. In Model 1, this predictor is synergistic with LAD exposure to an average dose above 32.7 Gy (OR=14.24, P=0.040), and in Model 2 with an anthracycline dose above 160 mg/m2 in the 3rd CHT course (OR=8.53, P=0.016). No synergistic effects of RT and CHT were obtained.
The decrease in EF of more than 10% during monitoring is linked to a preconditioning of cardiac function, as demonstrated by the pre-existence of certain changes in ECG patterns observed in the anterior leads, as well as alterations in T wave morphology recorded at these leads, as well as the posterior ones (see Figure 4). Moreover, this reduction of over 10% in EF is specifically associated with the administration of anti-HER2 therapy. It corresponds to the start of a declining trend following CHT as mentioned earlier (see Figure 3), and is also observed following RT or the initiation of anti-HER2 therapy, resulting in an initial decrease in EF of more than 5%.
By systematically exploring all combinations of parameters listed in Figure 4, a single statistically significant multinomial logistic model was obtained that includes as predictors of EF deterioration by more than 10%: post-CHT EF deterioration (OR =, P =) and pre-existence of some changes of T wave at the diagnosis of oncological disease (OR =, P =). The other estimated parameters of this model can be found in Supplementary Table 13 in the Supplementary Materials.
The onset of a declining trend in EF along with a decrease of ≥10% in its value is also linked to the development or exacerbation of major vessel diseases, cardiomyopathies, or left ventricle conditions (Figure 5). Moreover, EF reductions of ≥10% are associated with the initiation or deterioration of valvular conditions or diastolic relaxation dysfunction.
CHANGES IN ECHO- AND ELECTROCARDIOGRAPHIC FINDINGS:
The key monitored echocardiographic events are the decrease in EF of more than 5%, deterioration, or the development of a new echocardiographically diagnosable condition (see Supplementary Tables 9 and 10 in the Supplementary Materials). Therefore, overall modifications during monitoring can arise from either the worsening of an existing echocardiographic pattern at the initial diagnosis or the emergence of new pathologies (see Figure 6). Regardless of the mechanism behind these overall modifications, they are linked to the administration of anti-HER2 therapy (following CHT and without RT), particularly in cases with high-grade cardiac pathologies upon diagnosis. Specifically, cardiomyopathies and left ventricular conditions present at the time of diagnosis worsen during or after treatment, while the presence of delayed relaxation during diastole at diagnosis is associated with the emergence of new changes in echocardiographic patterns. The occurrence of this subclinical dysfunction during monitoring (see Supplementary Figure 5 in Supplementary Materials) is one of the most frequent events, often accompanied by valvular dysfunctions and various alterations in echocardiographic findings.
The occurrence of a ≥10% drop in EF during the monitoring period is linked to the emergence or exacerbation of echocardiographic findings indicative of the following types of pathologies: diastolic dysfunction (specifically delayed relaxation), cardiomyopathies or LV conditions, diseases affecting the great vessels, valvular diseases, and pericardial diseases (see Supplementary Figure 6 in the Supplementary Materials). The emergence or worsening of diastolic dysfunction is associated with the presence of pre-existing ECG findings, such as T wave modifications in posterior leads, as well as the administration of anti-HER2 therapy and CHT but not radiotherapy RT. The emergence or worsening of diseases affecting the great vessels, cardiomyopathies, and LV conditions is associated with distinct patterns of EF decline and the existence of at least 1 pre-existing cardiac condition. The presence of pre-existing cardiomyopathies and LV conditions is connected to the emergence or worsening of diseases affecting the great vessels, either during or after treatment. The emergence and exacerbation of valvular diseases are linked to the continuation of EF decline during treatment and the presence of pre-existing diastolic dysfunction (specifically delayed relaxation) or cardiomyopathies. Notably, the persistent negative progression of valvular diseases despite treatment is associated with the concurrent use of RT and anti-HER2 therapy (without CHT) at an average dose exceeding 8.1 Gy. This same treatment combination (RT and anti-HER2 therapy without CHT) and the presence of at least 1 existing cardiac condition are associated with the emergence of complications related to the pericardium.
Descriptive statistics of the time periods (in days, reported from the initiation of 1 of the therapies) elapsed until the onset of de novo or worsening of a pre-existing pathology are presented in Supplementary Table 14 in the Supplementary Materials. Moreover, the coexistence of various classes of these pathologies is observed (see Supplementary Figures 5–7 in the Supplementary Materials). Consequently, diastolic dysfunction characterized by delayed relaxation, which progresses over time, coexists with the continued evolution and emergence or exacerbation of valvular pathologies. Similarly, emerging or worsening cardiomyopathies and conditions affecting the left ventricle coexist with the development or worsening of large-vessel disease. These conditions, in turn, can coexist with pericardial complications or ongoing evolution of valvular pathologies.
As with echocardiographic findings, electrocardiographic findings can be altered due to the emergence of new changes or the exacerbation of existing ones at the time of diagnosis (refer to Table 5). Interestingly, no patterns of modification in the pre-existing ECG findings were identified. One of the biometric parameters linked to ECG changes is height (values above 1.64 m), and the most common ECG changes are associated with T wave morphology. Moreover, we observed alterations in ST junctions (J) or ST-segment depressions, which were correlated with the presence of thyroid disease, chronic infections, inflammatory conditions, or age exceeding 58 years. These modifications can be detected in both anterior and posterior ECG leads.
Discussion
RADIOTHERAPY TRIGGERS THE FIRST EF DROP >5%:
The EF shows a decrease of more than 5%, which may sometimes be irreversible, following RT (see Figure 2). However, within our study group, these drops demonstrated a continuous trend. They occurred after the initiation of anti-HER2 therapy because of the cumulative effects of the 3 non-surgical treatments. When assessing the direct impact of radiotherapy on the heart, it is essential to consider the doses received by the cardiac structures. This EF decreasing trend can be observed by considering the association between the maximum and mean doses received by the LAD and the heart, which are over 46.95 Gy and 32.7 Gy, and over 49.29 Gy and 8.12 Gy, respectively. The association’s OR and RR factors are identical, as these values are achieved simultaneously. A recent study indicated that maintaining the dose received by the left ventricular volume at 5 Gy and 10 Gy may not result in permanent cardiac dysfunction [38]. Typically, both the anterior heart and the LAD receive a dose that varies based on the patient’s anatomy. Our results are in agreement with other studies [39–42] which have also demonstrated that the mean dose of the heart and the volumes exposed to low doses serve as dependable indicators of RT-related cardiotoxic effects.
These findings seem to confirm the gradual development of RT-related cardiac events through early-onset, discreet ECG-detectable subclinical changes [43] which precede echocardiographic manifestations and changes in EF [44]. Some evidence underscores the potential of advanced echocardiographic techniques like tissue Doppler imaging (TDI) and speckle-tracking strain analysis, which can sensitively discern changes in myocardial velocities and intrinsic myocardial deformation, respectively, in the early detection and prediction of chemotherapy-induced cardiotoxicity, even before traditional measures such as LVEF indicate a functional decline [45].
After a prolonged period, these changes become clinically apparent. The specific patient-related factors that influence the time interval for the subclinical changes identified by ECG and/or echocardiography to progress into clinically evident cardiac adverse events remain uncertain. Our results suggest that radiation oncologists should initiate the monitoring of possible escalations of CV pathologies in the last quarter of the first year after RT. However, in patients without pre-existing cardiac conditions, EF lacks the sensitivity required to detect early left ventricular systolic dysfunction during breast cancer treatment. Conversely, global longitudinal strain (GLS) measured via speckle-tracking echocardiography is more sensitive in detecting subtle myocardial impairment [46,47].
These implications of dose-dependent cardiac pathologies are reported in the literature, particularly following RT in breast cancer and lymphomas [48,49]. However, the mechanisms through which RT damages cardiac tissue have not been fully elucidated. The specific regions of the heart that are most radiosensitive are unknown. Furthermore, no reference structures are included in the treatment plan to provide additional protection and consideration [50]. Defining the contour and volumes of cardiac and coronary structures independently from the literature can lead to significant variations. Therefore, it is necessary to propose a guide for delineating the heart structures in order to accurately estimate the doses received by those that are at a high risk for ischemic events [51].
There are still several unanswered questions regarding the cardiotoxic effects of RT. These include the identification of the radiosensitive cardiac structures involved, understanding the pathological mechanisms induced by irradiation, assessing the impact of low doses (less than 5 Gy), recognizing subclinical manifestations of functional cardiac modifications (detectable through echocardiography or ECG), determining enhancing factors such as age, weight, body mass index, cardiovascular comorbidities, and cardiotoxic chemotherapy. Advancements in these areas are essential to enhance post-therapeutic monitoring of patients and develop early diagnostic strategies for radiation-induced heart diseases. Reducing radiation, refining CV risk management, and early identification of CV issues are crucial for better outcomes [52].
In parallel with our findings, Yu et al [53] observed no early subclinical left ventricular (LV) dysfunction in HER2-positive breast cancer patients treated with sequential cardiotoxic therapies, using contemporary radiation techniques, emphasizing the push for safer radiation delivery methods like proton therapy to minimize heart exposure without compromising treatment efficacy. However, assessing the long-term cardiovascular benefits of such innovations remains challenging due to the extensive latency between initial radiation exposure and subsequent cardiac events, necessitating the exploration of intermediate biomarkers or surrogates to effectively gauge potential RT-related cardiac injuries.
CHEMOTHERAPY’S IMPACT ON EF CONTINUOUS DOWNWARD TREND:
The continuous decline in EF in relation to the initiation of CHT is associated, within our study group, with both RT, as indicated by the mean dose received by the left anterior descending artery (LAD), and CHT, characterized by a total dose exceeding 160 mg/m2 (considerably lower than the initially presumed toxic level). Additionally, the presence of pre-existing heart conditions at the time of diagnosis also contributes to this trend (see Figure 3).
This finding contradicts previous research that reported no acute cardiac toxicity (such as alterations in EF, troponin levels, or myoglobin drops) during the immediate weeks following CHT [54]. The absence of these factors, coupled with the presence of inflammatory conditions, is associated with an almost 3-fold increase in the time interval for recording such events, primarily in a subclinical manner. It is advisable that, in addition to the baseline cardiological evaluation before the initiation of CHT, there should be intensive cardiological monitoring (between administrations of CHT cycles) and possible cardioprotective treatment measures, especially in patients presenting these individual or cumulative risk factors.
Chemotherapy using anthracyclines (AC) [55] and taxanes has shown high effectiveness but carries a significant risk of cardiotoxicity [14,56,57], thus necessitating close monitoring of cardiac health [58]. These treatments induce apoptosis in cardiomyocytes, leading to fibrosis and asymptomatic reduction in EF soon after therapy [59]. The addition of taxanes to anthracycline-based regimens improves disease-free and overall survival in early breast cancer, while ongoing trials will determine the efficacy of taxane-based chemotherapy in HER2-negative early breast cancer [57]. This advancement may lead to the future replacement of anthracyclines by taxanes and novel regimens, owing to the lower cardiotoxicity associated with these drugs [57].
While EF has limitations in detecting and predicting cardiotoxicity in patients without pre-existing cardiac diseases at diagnosis, it is a valuable tool for assessing cardiotoxicity in the opposite scenario. A decrease in LV systolic strain after anthracycline treatment may indicate early myocardial impairment [58]. Myocardial damage of varying degrees, which can be inferred from an increase in troponin or myoglobin levels [54], is the underlying mechanism for EF reduction, particularly in patients who have received both ACs and RT.
Conversely, early detection of systolic global longitudinal strain (GLS), baseline end-systolic volume (ESV), or levels of blood biomarkers (such as ultrasensitive troponin I) are recognized as better predictors of major adverse cardiac events (MACE) in patients undergoing AC treatment. Implementing these measures on a large scale in clinical practice, however, is yet to be realized [14,56,58,60,61].
There is a lack of guidelines for the surveillance and prevention of treatment-induced cardiotoxicity in breast cancer survivors who have had limited exposure to ACs [62], or these guidelines are not widely implemented. However, secondary prevention should be personalized and implemented with caution [48]. It is recommended to increase cardiac surveillance, measure biomarkers, and administer cardioprotective therapy, although these actions may lead to medicalization and higher healthcare costs [62]. Additionally, implementing prophylactic measures towards the end of CHT could inform treatment decisions and mitigate cardiac adverse effects [14,60].
ANTI-HER2 THERAPY LEADS TO EF DROP >10% DURING MONITORING AND DRASTIC ECG MODIFICATIONS:
The initiation of a subclinical drop in EF after the completion of RT, as well as the consistent trend of EF decrease throughout the entire multimodal, non-surgical treatment period, indicates a pattern that is linked to reductions of more than 10% in EF (see Figure 6). Results of the HERA trial [63] show that a decrease in EF of more than 10% was reported in 9.18% of patients with left breast cancer who received RT, 8.99% of patients with right breast cancer who received RT, and 8.80% of patients who did not undergo RT. However, there was no statistically significant difference between these 3 groups (P=0.073) [63]. Our study shows that these reductions are typically irreversible and signify a significant deterioration in cardiac mechanical function. Conversely, existing modifications in T wave morphology in the anterior leads, and to a lesser extent in the posterior leads, are associated with this EF drop within a relatively short time, as categorized by the cardiotoxicity scale. Among all treatment approaches, anti-HER2 therapy, which is administered in HER2-positive patients [56,64], is most strongly correlated with this observed trend. Anti-HER2 therapy [56,64] increases the probability of echo/electrocardiographic changes starting in the second quarter of the first year in almost all patients for whom the values from which associations with these events begin are ubiquitous in the study group. It is noteworthy that the presence of such events starting 4 months after HER2 therapy is predictable for decreases in EF by 5% after RT, which confirms the cumulative cardiotoxic role of the 2 therapies.
Unfortunately, these therapies affect cardiac structures due to the presence of ErbB2/ErbB4 receptors in the myocardium. Trastuzumab (TRZ) further enhances the cardiotoxic effects of AC. Consequently, patients undergoing anthracycline-based chemotherapy (CHT) or anti-HER2 therapy, or both, may develop clinical heart failure with decreased EF [59,65,66]. Although the TRZ-induced cardiotoxic effect is sometimes partially reversible [56,64,67], careful evaluation and minimization of the risk of cardiac effect incidence are necessary, as AC and anti-HER2 therapies are associated with a risk of cardiotoxicity [68–71]. Therefore, the potential cardiac effects following CHT should be thoroughly assessed and minimized. During monitoring within our study group, a progressive decline of EF exceeding 10% was observed in individuals with pre-existing conditions affecting the great vessels and heart muscle (see Supplementary Figure 5 from the Supplementary Materials).
These results suggest that EF measurement should be utilized as a primary monitoring parameter for patients with a cardiac history who undergo multimodal treatment, including chemotherapy or anti-HER2 therapy. Conversely, ESV, GLS, and blood biomarkers should be employed for risk stratification.
An EF drop of more than 10% is associated with changes in electrocardiographic findings through 2 mechanisms: exacerbation of existing findings and the emergence of new findings, quantified as new electrocardiographic events. Among the treatments, anti-HER2 therapy is most strongly linked to the overall alteration in findings. Furthermore, when this therapy follows CHT without RT, the degree of association and the relative risk increase (see Supplementary Figure 6 from the Supplementary Materials).
Pre-existing conditions categorized as diseases of the great vessels worsen during treatment or have a higher likelihood of developing when there are existing heart muscle pathologies at the time of diagnosis. Other studies have also shown an increase in mitral valve regurgitation throughout the treatment, particularly during TRZ [72]. In this regard, our study shows that combinations are linked to particular cardiovascular conditions. Indeed, our findings show that the risk of valvular diseases persisting and progressing despite medical treatment rises when radiotherapy is combined with anti-HER2 therapy, especially when the average heart doses exceed 8.1 Gy (as seen in Supplementary Figure 6 from the Supplementary Materials). We also observed that the administration of CHT along with anti-HER2 therapy (without RT) is linked to delayed relaxation during diastole and pericardial diseases. Conversely, combining RT with anti-HER2 therapy negatively affects response to treatment of valvular diseases.
The TRZ/anthracycline/taxane regimen is beneficial for HER2+ patients but carries a significant cardiotoxicity risk [15,57,73], with it being relatively common but often asymptomatic [73]. Anthracycline treatment leads to a decline in LV and RV functions [72], but no further deterioration is observed during adjuvant TRZ treatment [72]. TRZ improves prognosis but can also cause LV cardiotoxicity in HER2-positive breast cancer patients. Additionally, it sometimes exhibits a global and uniform effect on myocardial function, as both ventricles demonstrate a similar degree and temporal pattern of impairment during TRZ treatment [74]. LV dilation and subclinical impairment persist for more than 2 years after treatment in women treated with ACs and TRZ [64]. Taxane/TRZ without ACs is a widely accepted adjuvant regimen for HER2+ breast cancer, as taxanes have surpassed ACs due to concerns of cardiotoxicity [57].
In patients without a history of cardiac events, both the mean and individual EFs remain stable during and after oncologic treatment, while GLS shows significant variation in several heart segments [72,75] during treatment. These variations are either fully or partially reversible after the completion of adjuvant treatment [46]. Furthermore, these alterations are not associated with significant cardiotoxicity or the late development of decreased EF [46].
However, we have observed that pre-existing cardiovascular diseases, particularly those affecting the cardiac muscle in the left ventricle, worsen under multimodal treatment that includes anti-HER2 therapy. These pre-existing cardiac conditions are linked to the development of new ones (see Figure 6). The lack of significant recovery [64] may be attributed to cardiac damage and remodelling.
The emergence of diastolic dysfunction, specifically delayed relaxation, is associated with subclinical alterations in the T wave morphology, particularly in the posterior leads. This indicates a discreet preconditioning of mechanical dysfunction through changes in the electrical function during the repolarization phase (see Supplementary Figure 6 from the Supplementary Materials). Additionally, ESV, which is related to this delayed relaxation, serves as an independent predictor of cardiotoxicity and is more reliable than EF [73]. However, diastolic strain rates determined by Fourier transform cardiac magnetic resonance (FT-CMR) may not be effective in monitoring subclinical TRZ-related myocardial dysfunction [75].
The occurrence of cardiomyopathies and LV conditions is associated with a continuous and progressive decline in mechanical function (with a drop in EF exceeding 10%) after each stage of the multimodal treatment.
LIMITATIONS OF THE STUDY:
Several considerations must be taken into account when interpreting our study’s findings. The retrospective design of our study and the relatively small study group may limit our ability to establish causal relationships and generalizability. Although efforts were made to standardize data collection, variations in the measurement of cardiac parameters, such as echocardiographic and ECG results, could introduce inconsistencies. The relatively modest sample size, while consistent with similar studies, might affect the statistical power and limit the broader applicability of our results. Additionally, the lack of external validation and the absence of systematic, equal follow-up periods for all patients could have influenced the accuracy of our findings. Certain clinical parameters, such as weight and those related to weight (BMI and BSA), were not dynamically measured during monitoring to assess their impact on variation. The relatively wide range of cardiovascular pathologies (ie, study group heterogeneity at the time of oncological disease diagnosis) rendered multivariable modelling of cardiac events unfeasible.
Despite these limitations, our study contributes to the understanding of cardiovascular events in the context of breast cancer treatment. Future research with larger cohorts and more controlled study designs could provide a more comprehensive assessment of these relationships.
Conclusions
Our study emphasizes the importance of considering patient factors, such as age, obesity, and comorbidities, as well as treatment factors, when predicting the occurrence and timing of subclinical ECG or echocardiographic events after chemotherapy and anti-HER2 therapy. Additionally, our findings suggest that different age limits may be associated with the occurrence of these events after chemotherapy compared to radiotherapy. Radiation therapy, anti-HER2 therapy, and their cumulative effects can lead to a slight but sometimes irreversible decrease in EF, which is linked to specific doses received by the LAD and the heart. Our study shows the importance of monitoring EF throughout the multimodal, non-surgical treatment period, particularly after RT and during anti-HER2 therapy. We observed that EF drops of more than 5% shortly after the completion of RT, which are accompanied by ECG changes involving T wave morphology, may indicate increased radiation doses to the LAD and whole heart, especially when they occur after the initiation of anti-HER2 therapy.
A decrease of more than 10% in EF may indicate a significant deterioration in mechanical cardiac function, often irreversible, particularly in patients with pre-existing cardiovascular diseases. We demonstrated that an EF drop >10% is associated with changes in electrocardiographic findings, and anti-HER2 therapy has the strongest association with overall changes in these features. Furthermore, we found that pre-existing cardiovascular diseases worsen under multimodal treatment, including anti-HER2 therapy, highlighting the need for careful monitoring and management of cardiac conditions in breast cancer patients undergoing this treatment.
One of the possible explanations for the impossibility of obtaining systematic multinomial logistic models containing 2 or more predictors of post-treatment cardiac function changes is the very high variability within the chosen sample. Overall, our results show that cardiac monitoring must be customized according to the presence of 1 or more risk factors in breast cancer patients. The reference point in establishing the moment of initiation of cardiological monitoring is the onset of each of the treatments that are part of the multimodal therapeutic strategy. Hence, there is a need for coherence in the interdisciplinary team and a rigorous communication of the post-therapeutic cardiac status within this team, which must include a cardio-oncologist and which must initiate cardioprotective measures of various kinds [62] in patients with non-negligible cardiac risk.
The accumulation of these variables in predictive multiparametric risk models for dramatic declines in EF should be studied in cohorts based on inclusion criteria formulated according to cut-off values or specific values of these variables. Therefore, large prospective studies are required to establish high-sensitivity cardiologic monitoring and non-invasive imaging as a means of cancer screening and surveillance in asymptomatic patients. These studies would enable targeted screening, follow-up, and intervention in a systematic, high-frequency, and personalized manner, especially in the period immediately following the initiation of a multimodal treatment strategy. For individuals with irradiated left-side breast cancer, cardiac investigations like high-precision ECG and echocardiography should be implemented as the standard of care, along with the development of monitoring guidelines. Additionally, due to the lack of clinical awareness of such pathological advancements, ongoing research in this field and the concurrent reporting of data from specialized literature are imperative.
Figures
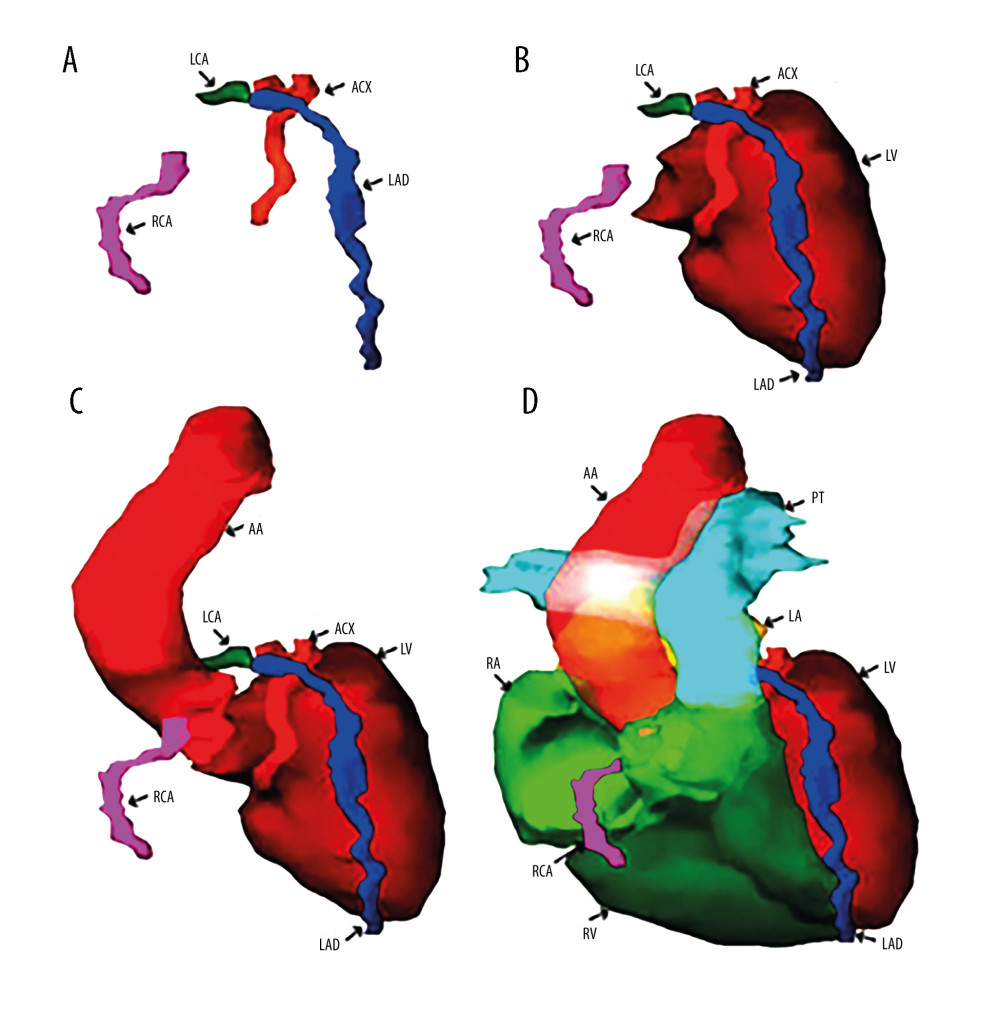
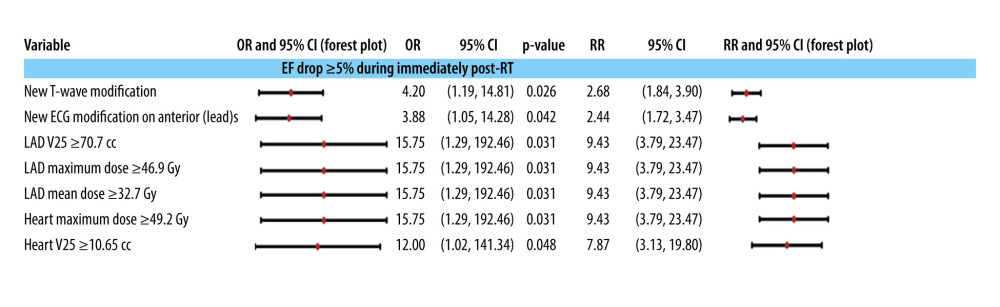
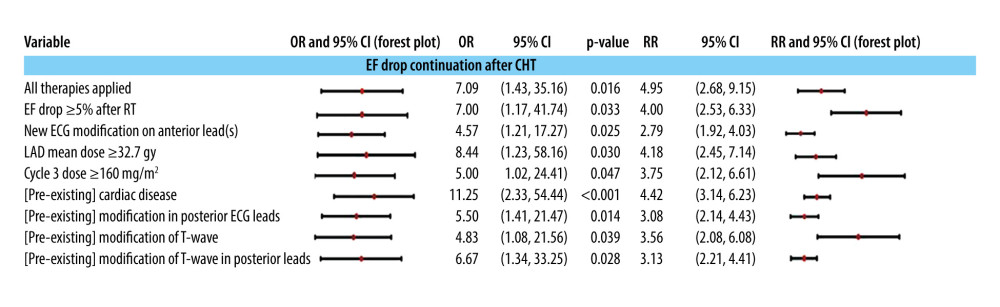
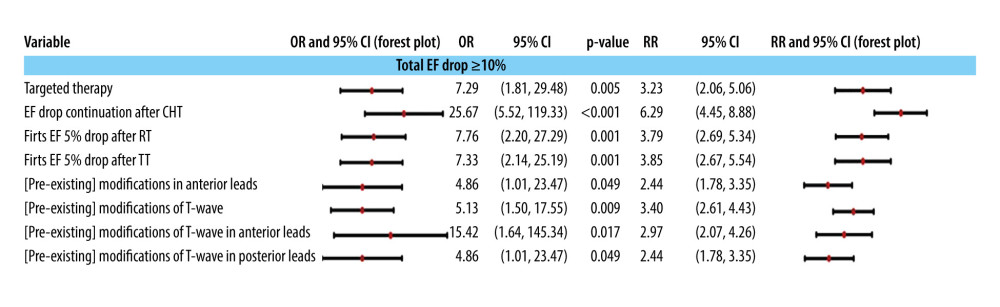
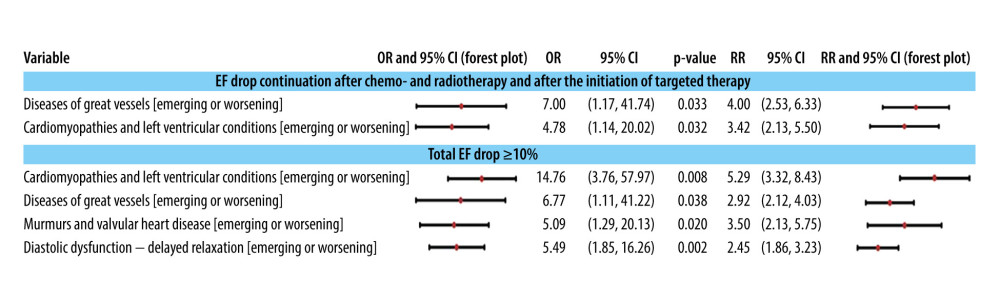
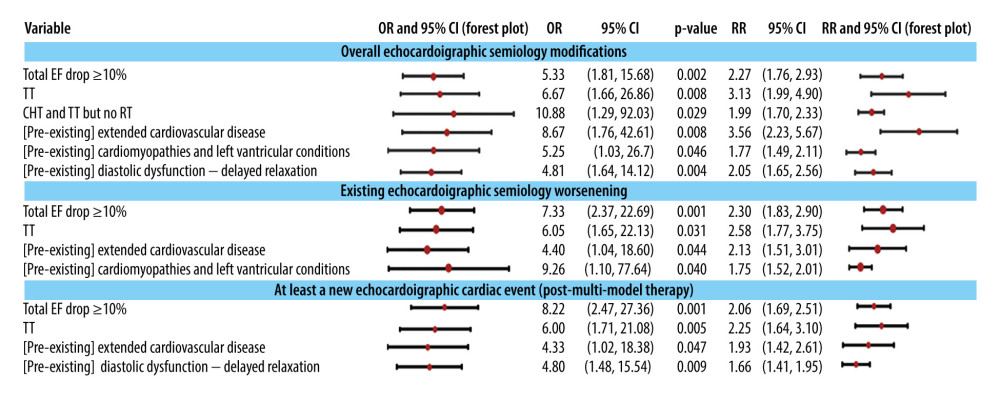
Tables
Table 1. Classes of variables used in this study.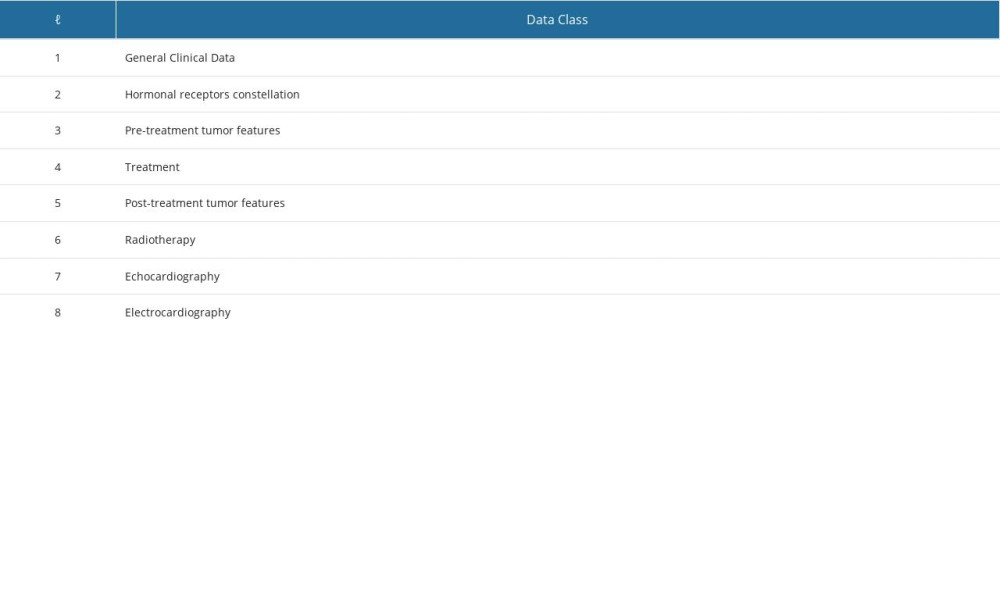
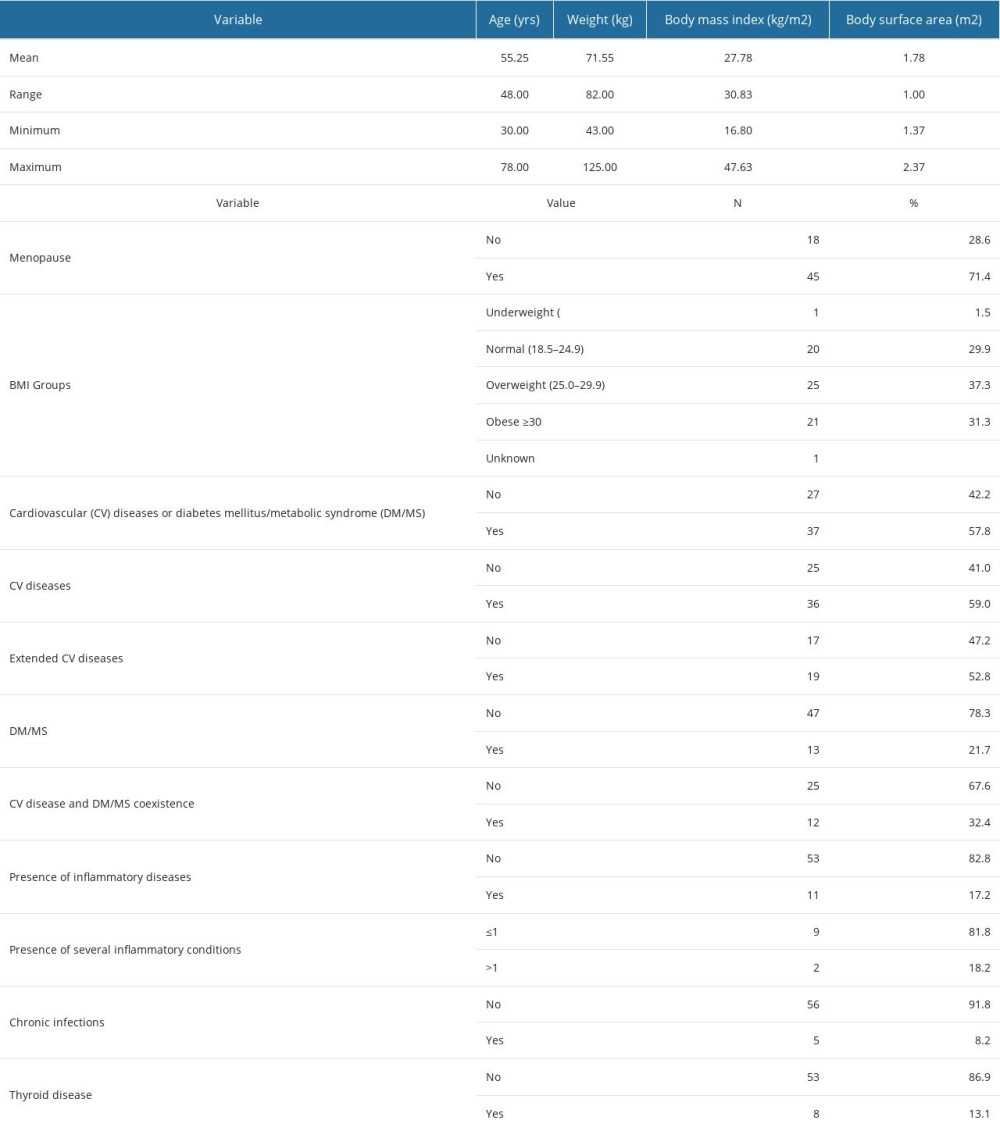
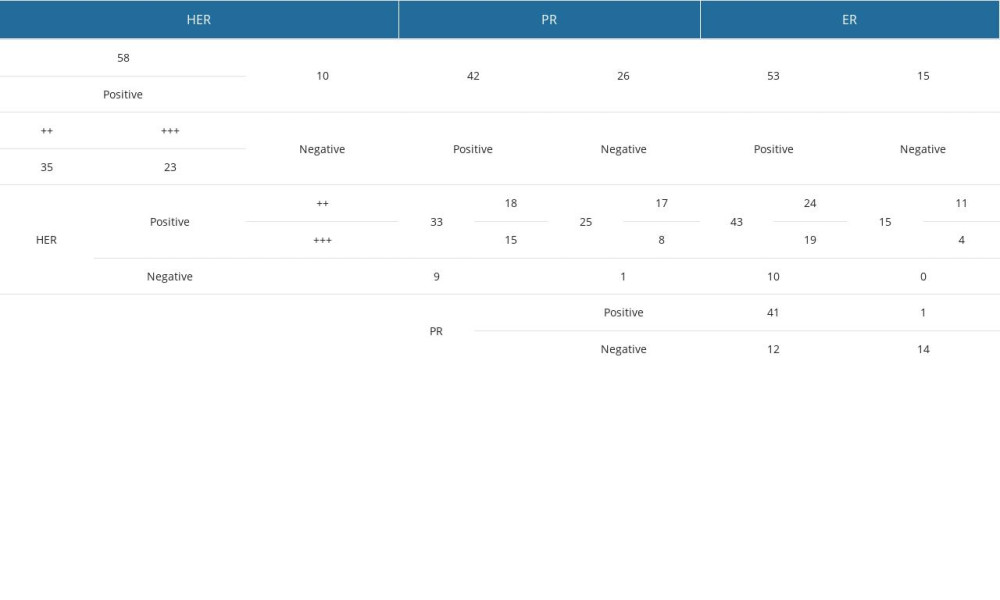
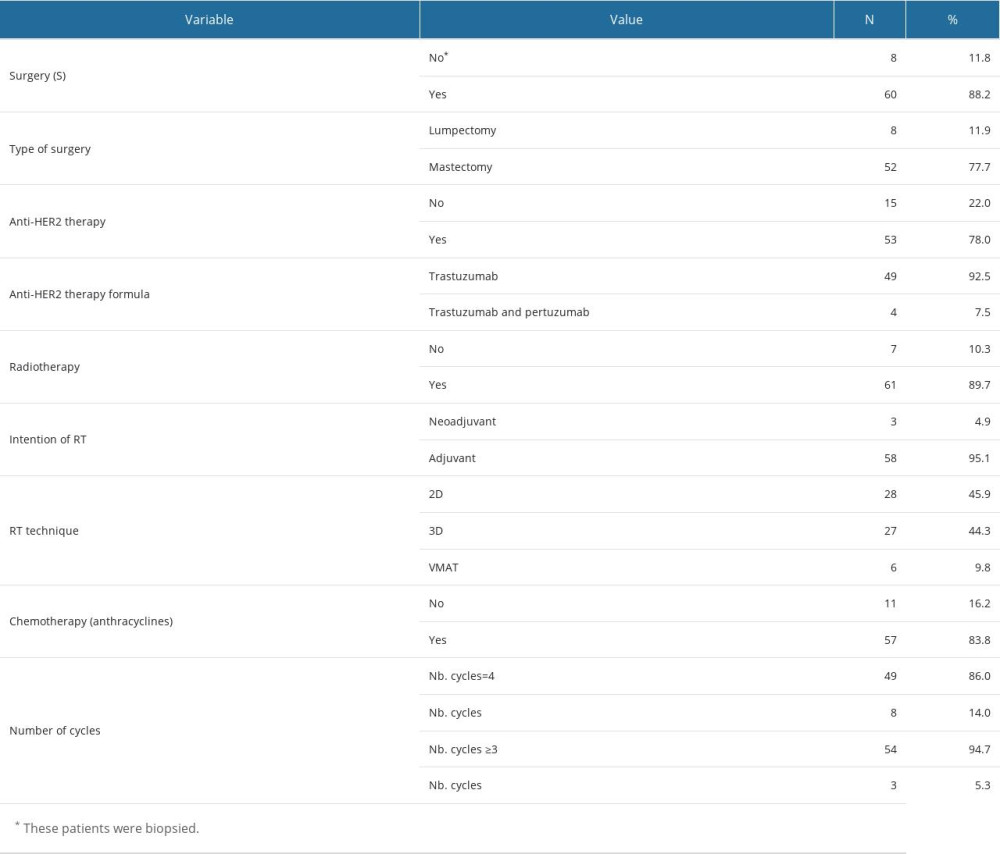
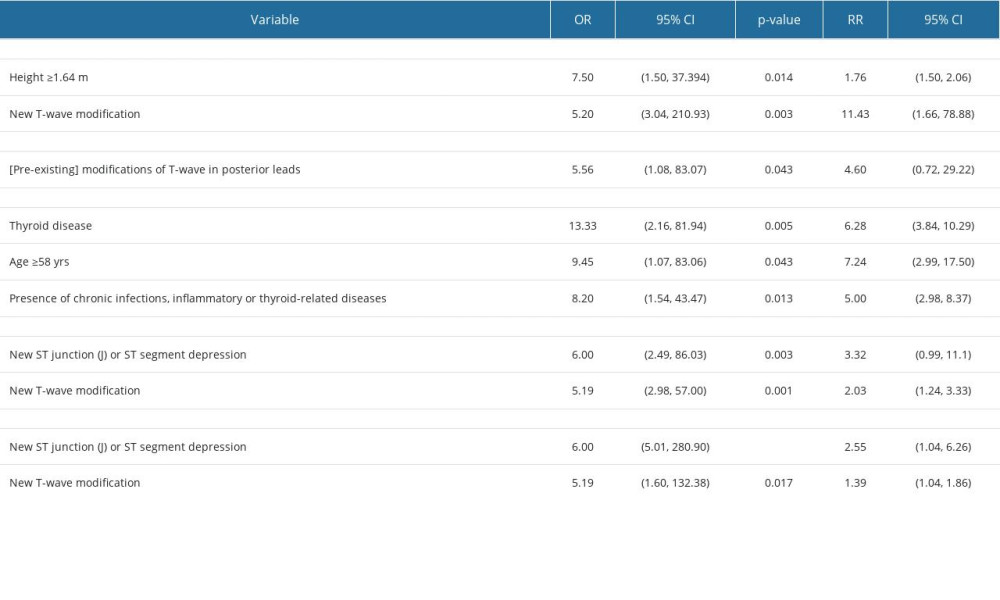
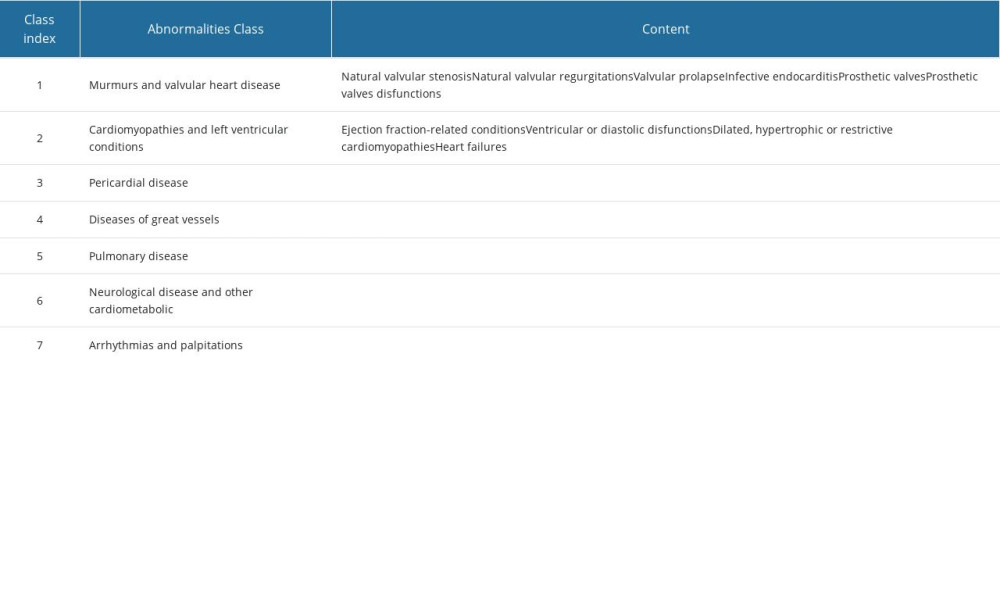
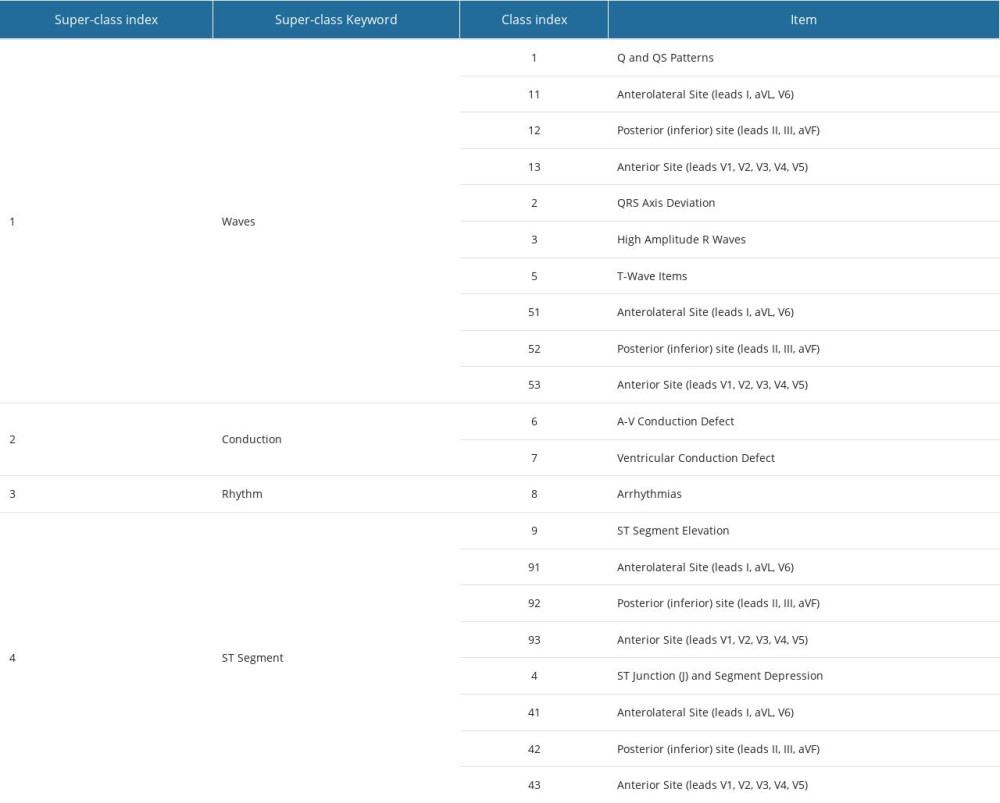
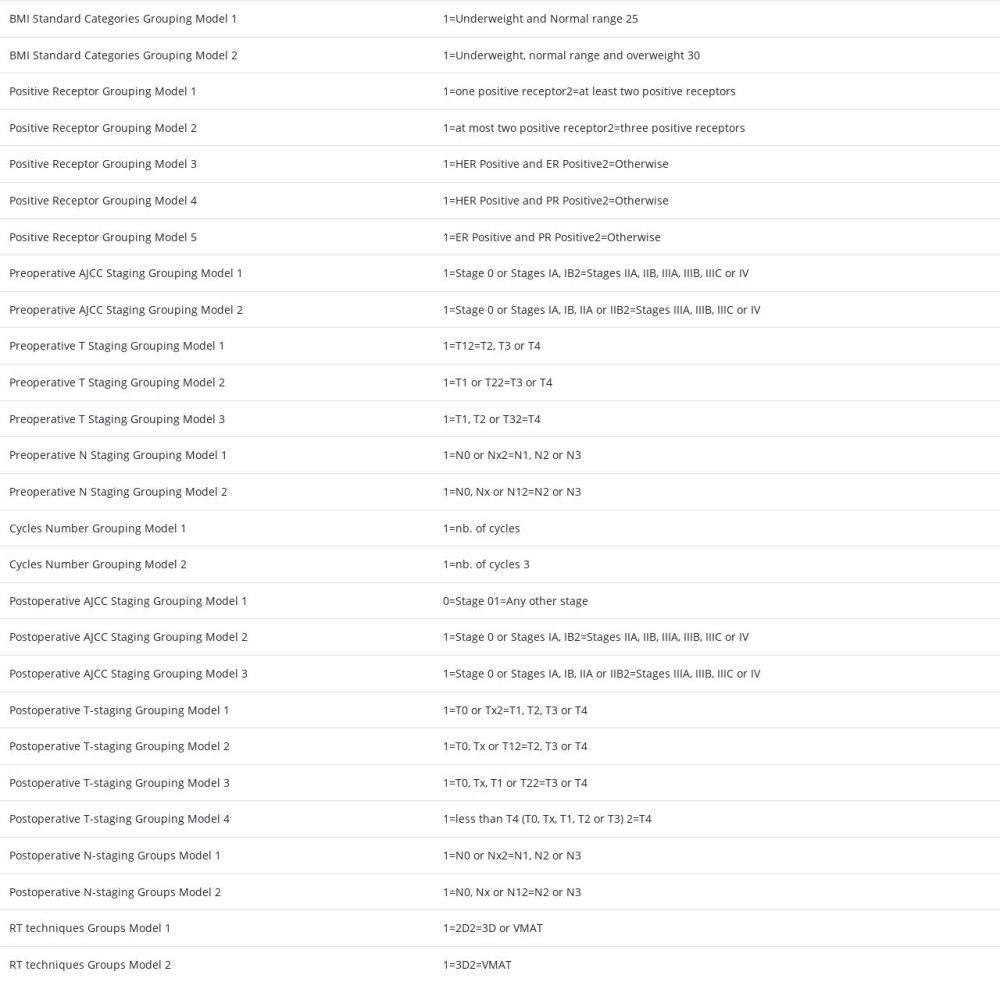
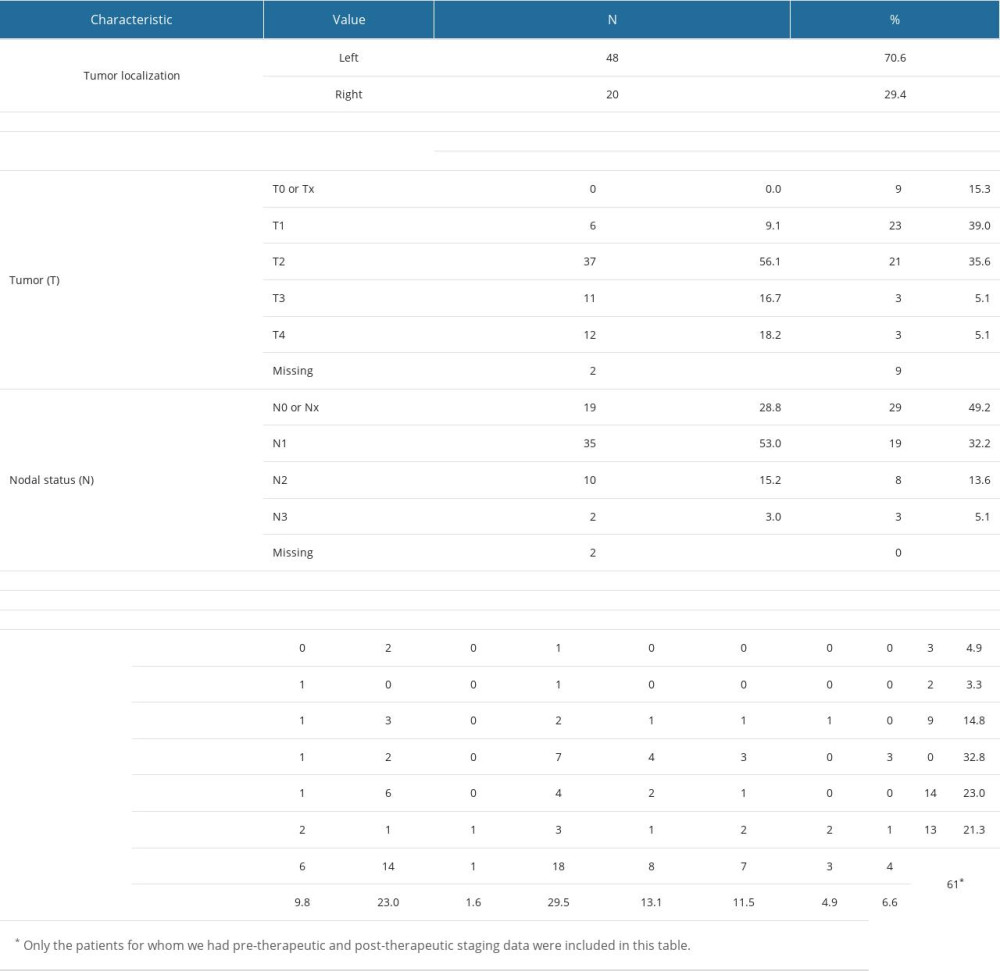
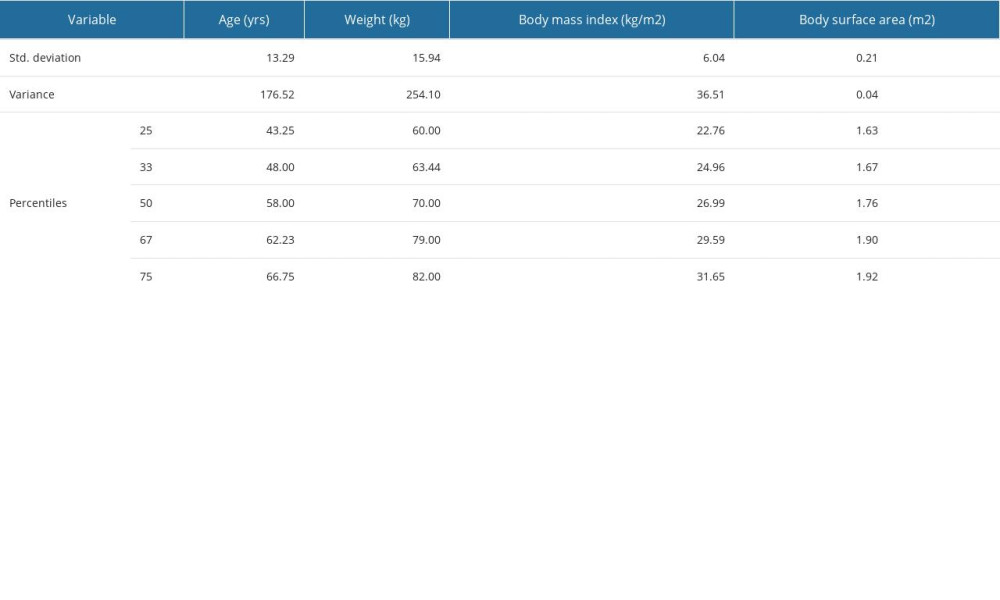
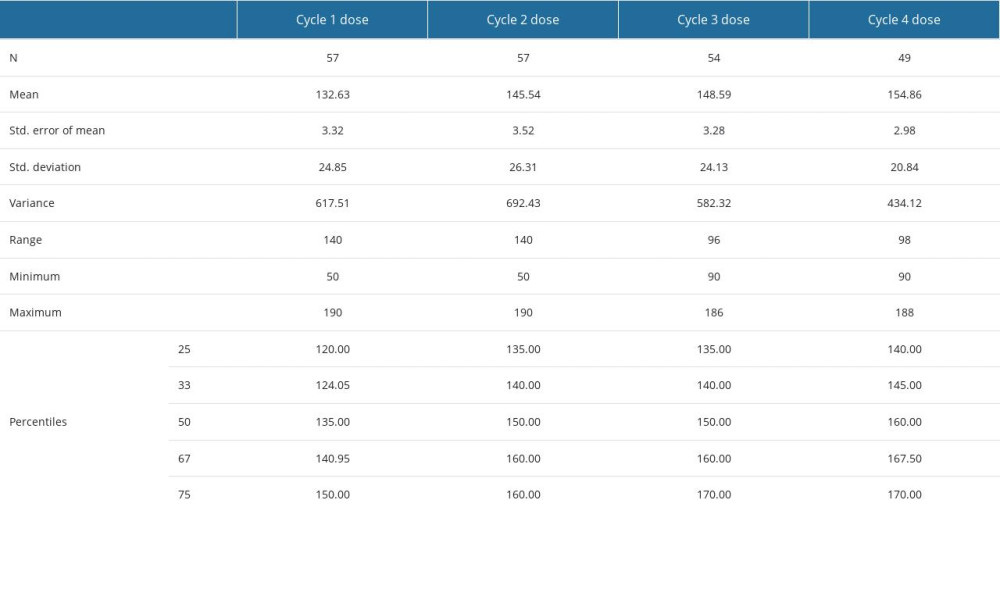
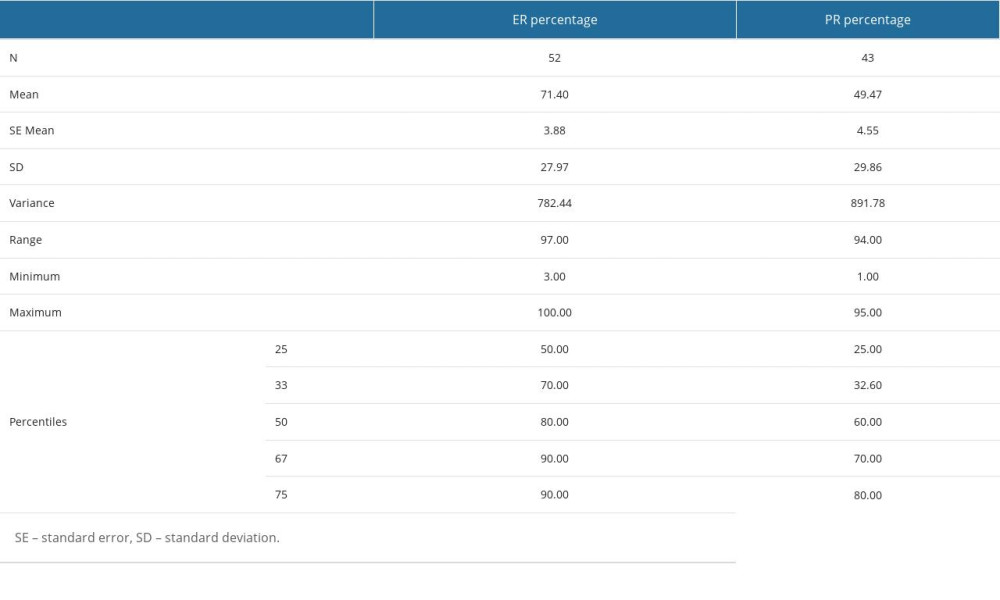
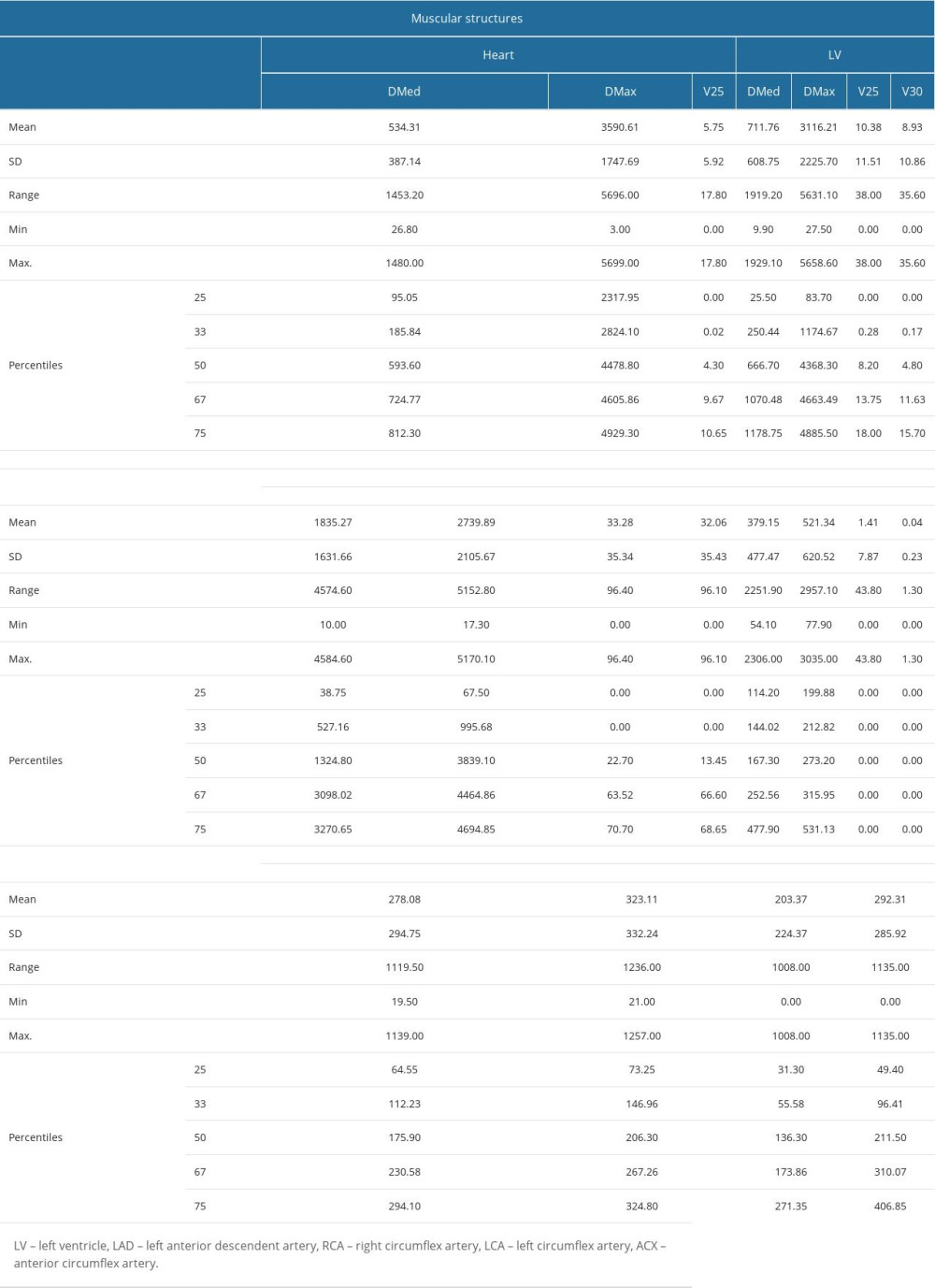
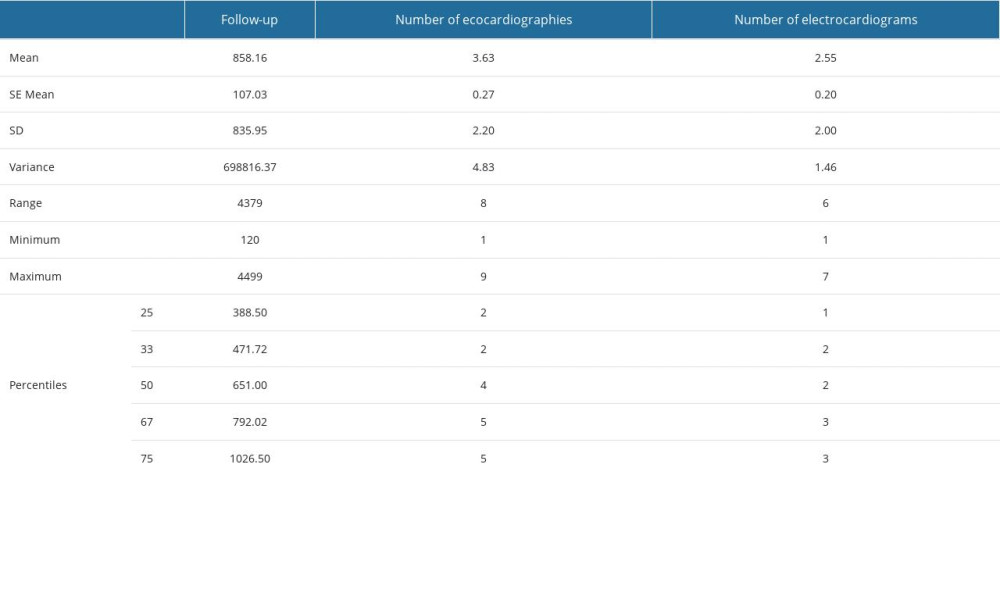
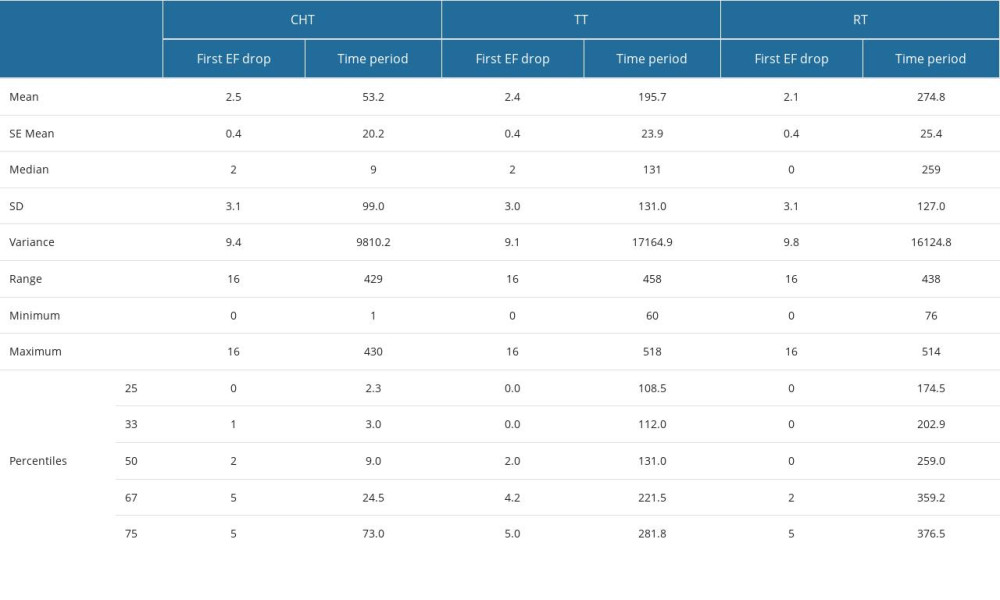
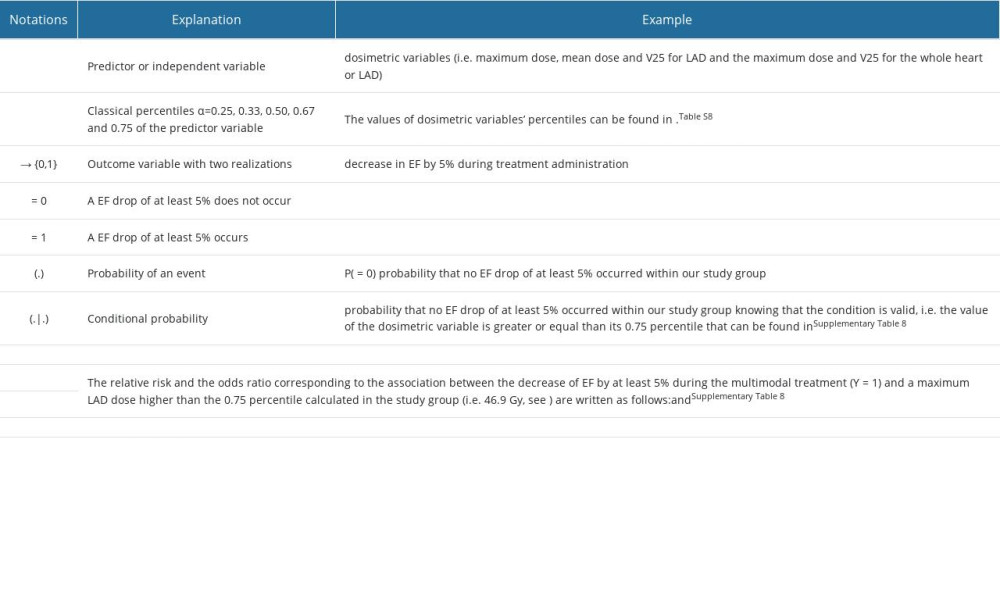
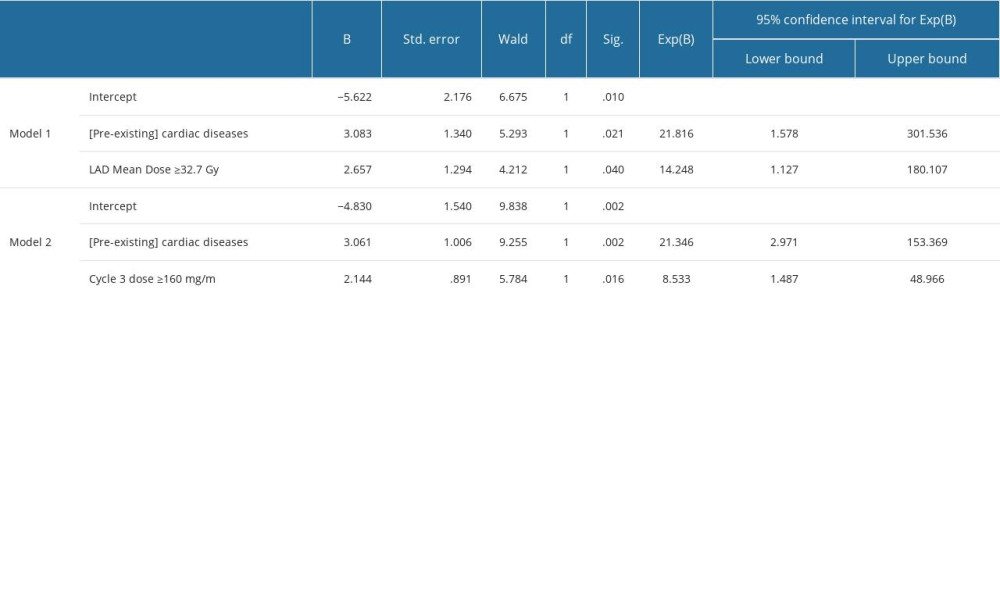
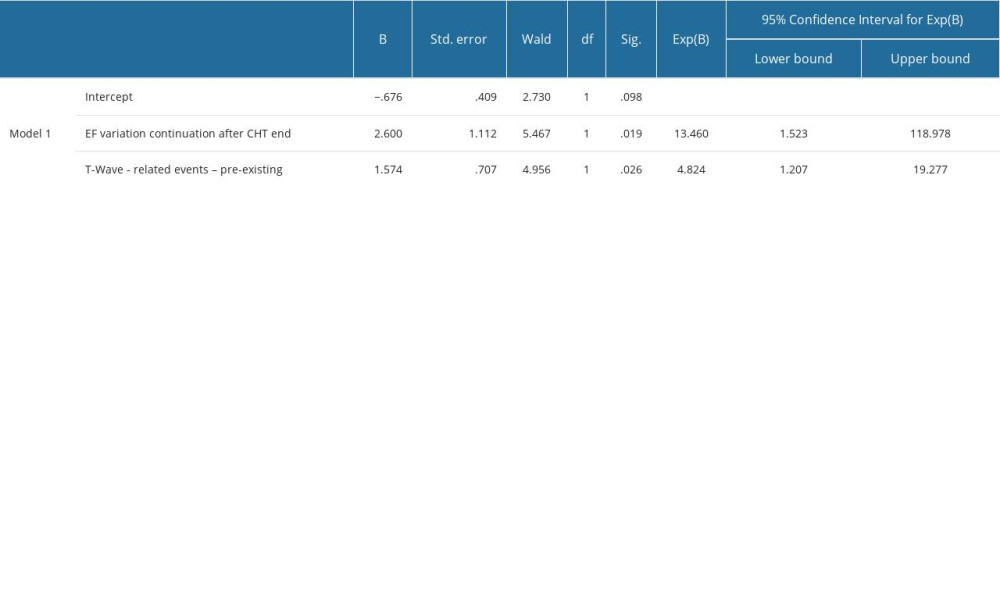
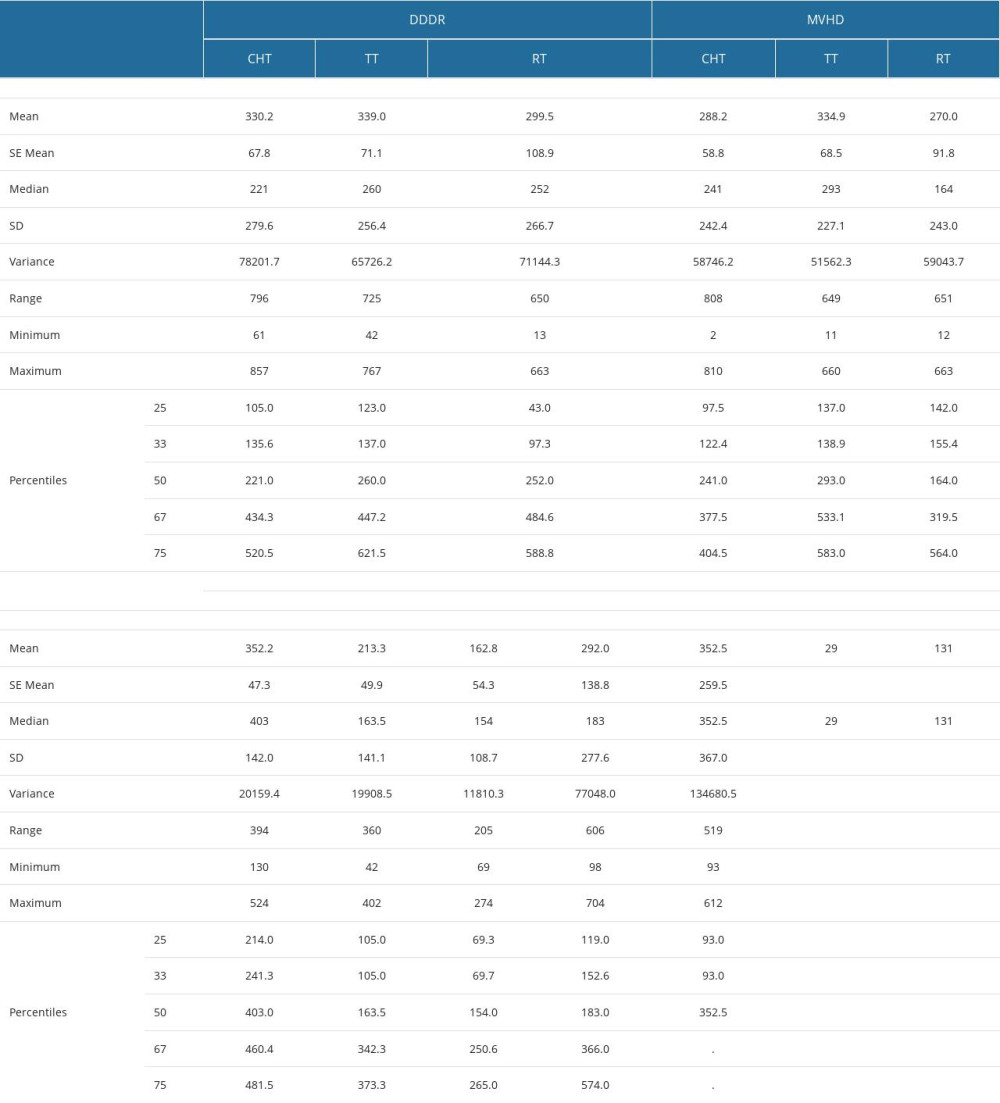
References
1. Mery B, Fouilloux A, Rowinski E, Cardiovascular disease events within 5 years after a diagnosis of breast cancer: BMC Cancer, 2020; 20(1); 337
2. Díaz-Gavela A, Figueiras-Graillet L, Luis Á, Breast radiotherapy-related cardiotoxicity. When, how, why. risk prevention and control strategies: Cancers, 2021; 13(7); 1712
3. Bhave M, Shah AN, Akhter N, Rosen ST, An update on the risk prediction and prevention of anticancer therapy-induced cardiotoxicity: Curr Opin Oncol, 2014; 26(6); 590-99
4. : Home-Eurostat https://ec.europa.eu/eurostat
5. , Left breast radiation therapy doubles heart disease risk in young women: Oncol Times, 2021; 43(21); 25-25
6. Papageorgiou C, Andrikopoulou A, Dimopoulos MA, Zagouri F, Cardiovascular toxicity of breast cancer treatment: An update: Cancer Chemother Pharmacol, 2021; 88(1); 15-24
7. Zhang M, Yang H, Xu C, Risk factors for anthracycline-induced cardiotoxicity in breast cancer treatment: A meta-analysis: Front Oncol, 2022; 12; 899782
8. Curigliano G, Cardinale D, Suter T, Cardiovascular toxicity induced by chemotherapy, targeted agents and radiotherapy: ESMO Clinical Practice Guidelines: Ann Oncol, 2012; 23; vii155-vii66
9. An D, Choi J, Lee J, Time to surgery and survival in breast cancer: BMC Surg, 2022; 22(1); 388
10. De Angelis R, Sant M, Coleman MP, Cancer survival in Europe 1999–2007 by country and age: Results of EUROCARE-5 – a population-based study: Lancet Oncol, 2014; 15(1); 23-34
11. McGowan JV, Chung R, Maulik A, Anthracycline chemotherapy and cardiotoxicity: Cardiovasc Drugs Ther, 2017; 31(1); 63-75
12. Douedi S, Carson MP, Anthracycline medications (doxorubicin): StatPearls, 2023, StatPearls Publishing http://www.ncbi.nlm.nih.gov/books/NBK551633/
13. Mohan N, Jiang J, Dokmanovic M, Wu WJ, Trastuzumab-mediated cardiotoxicity: Current understanding, challenges, and frontiers: Antib Ther, 2018; 1(1); 13-17
14. Sawaya H, Sebag IA, Plana JC, Assessment of echocardiography and biomarkers for the extended prediction of cardiotoxicity in patients treated with anthracyclines, taxanes, and trastuzumab: Circ Cardiovasc Imaging, 2012; 5(5); 596-603
15. Jerusalem G, Lancellotti P, Kim SB, HER2+ breast cancer treatment and cardiotoxicity: Monitoring and management: Breast Cancer Res Treat, 2019; 177(2); 237-50
16. Ghosh AK, Walker JM, Cardio-Oncology – a new subspecialty with collaboration at its heart: Indian Heart J, 2017; 69(4); 556-62
17. Brown LC, Mutter RW, Halyard MY, Benefits, risks, and safety of external beam radiation therapy for breast cancer: Int J Womens Health, 2015; 7; 449-58
18. Mrotzek SM, Rassaf T, Totzeck M, Cardiovascular damage associated with chest irradiation: Front Cardiovasc Med, 2020; 7; 41
19. Lewis GD, Farach A, Cardiovascular toxicities of radiation therapy: Methodist DeBakey Cardiovasc J, 2019; 15(4); 274-81
20. Rammohan N, Randall JW, Yadav P, History of technological advancements towards MR-Linac: The future of image-guided radiotherapy: J Clin Med, 2022; 11(16); 4730
21. Chitapanarux I, Nobnop W, Tippanya D, Clinical outcomes and dosimetric study of hypofractionated Helical TomoTherapy in breast cancer patients: PLoS One, 2019; 14(1); e0211578
22. Freislederer P, Kügele M, Öllers M, Recent advances in surface guided radiation therapy: Radiat Oncol, 2020; 15(1); 187
23. Hui SK, Das RK, Kapatoes J, Helical tomotherapy as a means of delivering accelerated partial breast irradiation: Technol Cancer Res Treat, 2004; 3(6); 639-46
24. Patil S, Pingle SR, Shalaby K, Kim AS, Mediastinal irradiation and valvular heart disease: Cardio-Oncol, 2022; 8(1); 7
25. Wiedemann J, Coppes RP, Van Luijk P, Radiation-induced cardiac side-effects: The lung as target for interacting damage and intervention: Front Oncol, 2022; 12; 931023
26. Lancellotti P, Nkomo VT, Badano LP, Expert consensus for multi-modality imaging evaluation of cardiovascular complications of radiotherapy in adults: A report from the European Association of Cardiovascular Imaging and the American Society of Echocardiography: Eur Heart J Cardiovasc Imaging, 2013; 14(8); 721-40
27. Koh J, Kim MJ, Introduction of a new staging system of breast cancer for radiologists: An emphasis on the prognostic stage: Korean J Radiol, 2019; 20(1); 69-82
28. Cheitlin MD, Alpert JS, Armstrong WF, ACC/AHA guidelines for the clinical application of echocardiography: Circulation, 1997; 95(6); 1686-44
29. Surawicz B, Childers R, Deal BJ, Gettes LS, AHA/ACCF/HRS recommendations for the standardization and interpretation of the electrocardiogram: Circulation, 2009; 119(10); e235-e40
30. Prineas RJ, Crow RS, Zhang ZM: The Minnesota Code Manual of Electrocardiographic Findings: Including measurement and comparison with the novacode: standards and procedures for ECG measurement in epidemiologic and clinical trails. 2nd ed., new and enlarged, 2010, Springer
31. Early Breast Cancer Trialists’ Collaborative Group (EBCTCG), Long-term outcomes for neoadjuvant versus adjuvant chemotherapy in early breast cancer: Meta-analysis of individual patient data from ten randomised trials: Lancet Oncol, 2018; 19(1); 27-39
32. Ahn S, Woo JW, Lee K, Park SY, HER2 status in breast cancer: Changes in guidelines and complicating factors for interpretation: J Pathol Transl Med, 2020; 54(1); 34-44
33. Piccart-Gebhart MJ, Procter M, Leyland-Jones B, Trastuzumab after adjuvant chemotherapy in HER2-positive breast cancer: N Engl J Med, 2005; 353(16); 1659-72
34. Gentile MS, Usman AA, Neuschler EI, Contouring guidelines for the axillary lymph nodes for the delivery of radiation therapy in breast cancer: Evaluation of the RTOG breast cancer atlas: Int J Radiat Oncol, 2015; 93(2); 257-65
35. Fontanilla HP, Woodward WA, Lindberg ME, Current clinical coverage of Radiation Therapy Oncology Group-defined target volumes for postmastectomy radiation therapy: Pract Radiat Oncol, 2012; 2(3); 201-9
36. Feng M, Moran JM, Koelling T, Development and validation of a heart atlas to study cardiac exposure to radiation following treatment for breast cancer: Int J Radiat Oncol Biol Phys, 2011; 79(1); 10-18
37. Lyon AR, López-Fernández T, Couch LS, 2022 ESC Guidelines on cardio-oncology developed in collaboration with the European Hematology Association (EHA), the European Society for Therapeutic Radiology and Oncology (ESTRO) and the International Cardio-Oncology Society (IC-OS): Eur Heart J, 2022; 43(41); 4229-61
38. Abraham A, Sanghera KP, Gheisari F, Is radiation-induced cardiac toxicity reversible? Prospective evaluation of patients with breast cancer enrolled in a phase 3 randomized controlled trial: Int J Radiat Oncol Biol Phys, 2022; 113(1); 125-34
39. Jensen CA, Funderud M, Lervåg C, Free breathing VMAT versus deep inspiration breath hold 3D conformal radiation therapy for early stage left sided breast cancer: J Appl Clin Med Phys, 2021; 22(4); 44-51
40. Jin GH, Chen LX, Deng XW, A comparative dosimetric study for treating left-sided breast cancer for small breast size using five different radiotherapy techniques: Conventional tangential field, filed-in-filed, Tangential-IMRT, Multi-beam IMRT and VMAT: Radiat Oncol, 2013; 8(1); 89
41. Zheng H, Lai YQ, Zhou Y, A comparative dosimetric study of seven radiation techniques for breast cancer after mastectomy and immediate breast reconstruction: Transl Cancer Res, 2017; 6(4); 15303
42. Osman SOS, Hol S, Poortmans PM, Essers M, Volumetric modulated arc therapy and breath-hold in image-guided locoregional left-sided breast irradiation: Radiother Oncol, 2014; 112(1); 17-22
43. Tang S, Otton J, Holloway L, Quantification of cardiac subvolume dosimetry using a 17 segment model of the left ventricle in breast cancer patients receiving tangential beam radiotherapy: Radiother Oncol, 2019; 132; 257-65
44. Liang X, Wang Y, Yin X, Electrocardiographic characteristics of breast cancer patients treated with chemotherapy: Cardiol Res Pract, 2020; 2020; 6678503
45. Gulati G, Zhang KW, Scherrer-Crosbie M, Ky B, Cancer and cardiovascular disease: The use of novel echocardiography measures to predict subsequent cardiotoxicity in breast cancer treated with anthracyclines and trastuzumab: Curr Heart Fail Rep, 2014; 11(4); 366-73
46. Mele D, Malagutti P, Indelli M, Reversibility of left ventricle longitudinal strain alterations induced by adjuvant therapy in early breast cancer patients: Ultrasound Med Biol, 2016; 42(1); 125-32
47. Trivedi SJ, Choudhary P, Lo Q, Persistent reduction in global longitudinal strain in the longer term after radiation therapy in patients with breast cancer: Radiother Oncol, 2019; 132; 148-54
48. Bansal N, Adams MJ, Ganatra S, Strategies to prevent anthracycline-induced cardiotoxicity in cancer survivors: Cardio-Oncol, 2019; 5(1); 18
49. Clarke M, Collins R, Darby S, Effects of radiotherapy and of differences in the extent of surgery for early breast cancer on local recurrence and 15-year survival: An overview of the randomised trials: Lancet, 2005; 366(9503); 2087-106
50. Sardaro A, Petruzzelli MF, D’Errico MP, Radiation-induced cardiac damage in early left breast cancer patients: Risk factors, biological mechanisms, radiobiology, and dosimetric constraints: Radiother Oncol, 2012; 103(2); 133-42
51. Loap P, Tkatchenko N, Nicolas E, Optimization and auto-segmentation of a high risk cardiac zone for heart sparing in breast cancer radiotherapy: Radiother Oncol, 2020; 153; 146-54
52. Ganatra S, Chatur S, Nohria A, How to diagnose and manage radiation cardiotoxicity: JACC CardioOncology, 2020; 2(4); 655-60
53. Yu A, Ho A, Braunstein L, Assessment of early radiation-induced changes in LV function by myocardial strain imaging after breast radiation therapy: J Am Soc Echocardiogr, 2019; 32(4); 521-28
54. Nisticò C, Bria E, Carpino A, Evaluation of weekly epirubicin-paclitaxel (EP) cardiotoxicity with serum troponin-t and myoglobin and echocardiography in advanced breast cancer (ABC): J Clin Oncol, 2004; 22(14 Suppl); 775
55. Lorusso V, Giota F, Bordonaro R, Non-pegylated liposome-encapsulated doxorubicin citrate plus cyclophosphamide or vinorelbine in metastatic breast cancer not previously treated with chemotherapy: A multicenter phase III study: Int J Oncol, 2014; 45(5); 2137-42
56. wen Fei H, Ali MT, Tan TC, Left ventricular global longitudinal strain in HER-2 + breast cancer patients treated with anthracyclines and trastuzumab who develop cardiotoxicity is associated with subsequent recovery of left ventricular ejection fraction: Echocardiography, 2016; 33(4); 519-26
57. Greene J, Hennessy B, The role of anthracyclines in the treatment of early breast cancer: J Oncol Pharm Pract, 2015; 21(3); 201-12
58. Stoodley PW, Richards DAB, Hui R, Two-dimensional myocardial strain imaging detects changes in left ventricular systolic function immediately after anthracycline chemotherapy: Eur J Echocardiogr, 2011; 12(12); 945-52
59. Upshaw JN, Cardio-oncology: Protecting the heart from curative breast cancer treatment: Gland Surg, 2018; 7(4); 35065
60. Stoodley PW, Richards DAB, Boyd A, Left ventricular systolic function in HER2/neu negative breast cancer patients treated with anthracycline chemotherapy: A comparative analysis of left ventricular ejection fraction and myocardial strain imaging over 12 months: Eur J Cancer, 2013; 49(16); 3396-403
61. Mousavi N, Tan TC, Ali M, Halpern EF, Echocardiographic parameters of left ventricular size and function as predictors of symptomatic heart failure in patients with a left ventricular ejection fraction of 50–59% treated with anthracyclines: Eur Heart J Cardiovasc Imaging, 2015; 16(9); 977-84
62. Levis BE, Binkley PF, Shapiro CL, Cardiotoxic effects of anthracycline-based therapy: What is the evidence and what are the potential harms?: Lancet Oncol, 2017; 18(8); e445-e56
63. Bachir B, Anouti S, Abi Jaoude J, Evaluation of cardiotoxicity in HER-2-positive breast cancer patients treated with radiation therapy and trastuzumab: Int J Radiat Oncol, 2022; 113(1); 135-42
64. Tan TC, Bouras S, Sawaya H, Time trends of left ventricular ejection fraction and myocardial deformation indices in a cohort of women with breast cancer treated with anthracyclines, taxanes, and trastuzumab: J Am Soc Echocardiogr, 2015; 28(5); 509-14
65. Dent S, Morse A, Burnette S, Guha A, Moore H, Cardiovascular toxicity of novel HER2-targeted therapies in the treatment of breast cancer: Curr Oncol Rep, 2021; 23(11); 128
66. Criscitiello C, Metzger-Filho O, Saini KS, Targeted therapies in breast cancer: Are heart and vessels also being targeted?: Breast Cancer Res, 2012; 14(3); 1-15
67. Vinante L, Evangelista L, Lora O, EP-1015 Radiotherapy and adjuvant trastuzumab for HER-2 breast cancer: Clinical outcome and cardiotoxicity: Radiother Oncol, 2012; 103; S399
68. Jurcut R, Wildiers H, Ganame J, Detection and monitoring of cardiotoxicity-what does modern cardiology offer?: Support Care Cancer Off J Multinatl Assoc Support Care Cancer, 2008; 16(5); 437-45
69. Ewer MS, Vooletich MT, Durand JB, Reversibility of trastuzumab-related cardiotoxicity: New insights based on clinical course and response to medical treatment: J Clin Oncol, 2005; 23(31); 7820-26
70. Perez EA, Suman VJ, Davidson NE, Cardiac safety analysis of doxorubicin and cyclophosphamide followed by paclitaxel with or without trastuzumab in the North Central Cancer Treatment Group N9831 Adjuvant Breast Cancer Trial: J Clin Oncol, 2008; 26(8); 1231-38
71. Moja L, Tagliabue L, Balduzzi S, Trastuzumab containing regimens for early breast cancer: Cochrane Database Syst Rev, 2012(4); CD006243
72. Lange SA, Jung J, Jaeck A, Subclinical myocardial impairment occurred in septal and anterior LV wall segments after anthracycline-embedded chemotherapy and did not worsen during adjuvant trastuzumab treatment in breast cancer patients: Cardiovasc Toxicol, 2016; 16(2); 193-206
73. Bergamini C, Torelli F, Ghiselli L, Left ventricular end-diastolic volume as early indicator of trastuzumab-related cardiotoxicity in HER2+ breast cancer patients: results from a single-center retrospective study: Minerva Cardiolangiol, 2017; 65(3); 278-87
74. Keramida K, Farmakis D, Bingcang J, Longitudinal changes of right ventricular deformation mechanics during trastuzumab therapy in breast cancer patients: Eur J Heart Fail, 2019; 21(4); 529-35
75. Gong IY, Ong G, Brezden-Masley C, Early diastolic strain rate measurements by cardiac MRI in breast cancer patients treated with trastuzumab: A longitudinal study: Int J Cardiovasc Imaging, 2019; 35(4); 653-62
Figures
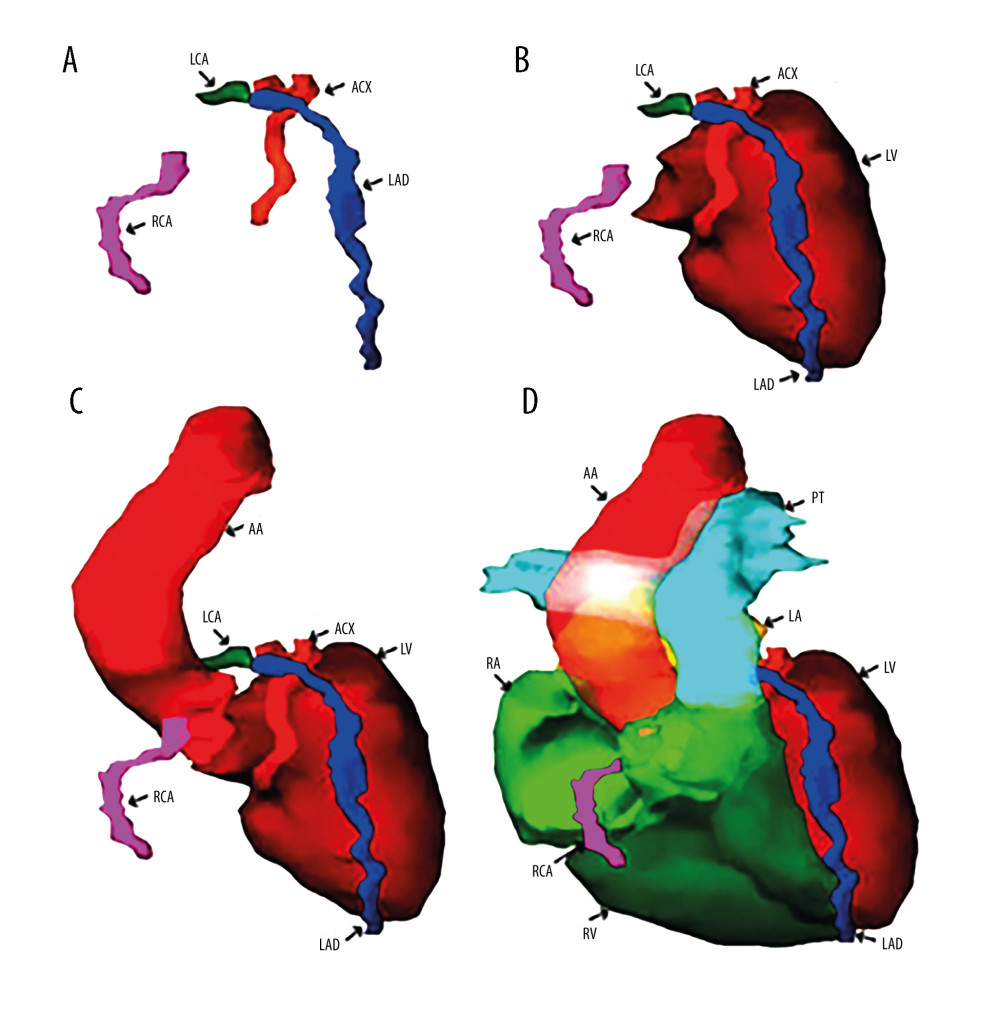
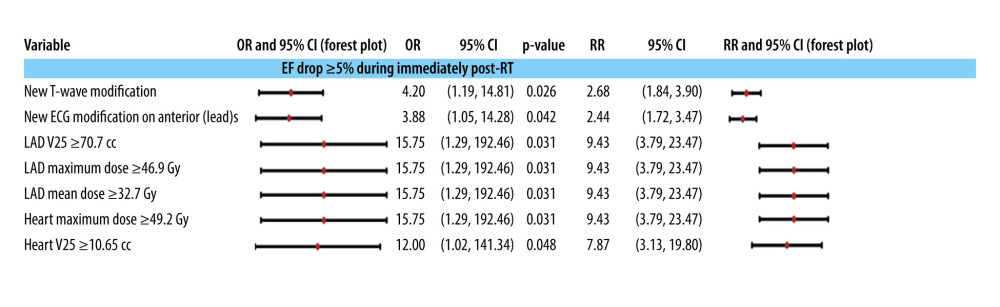
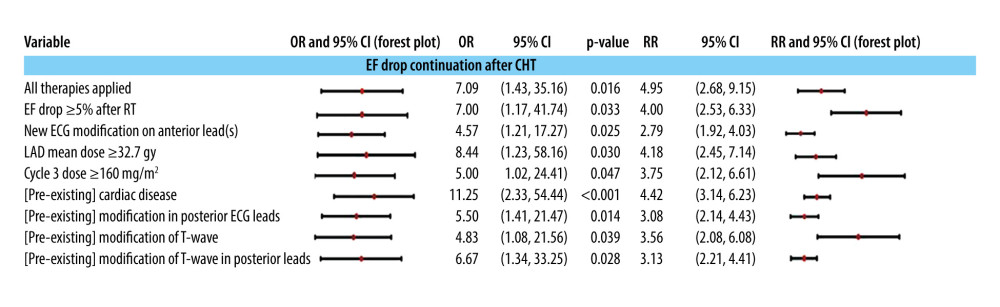
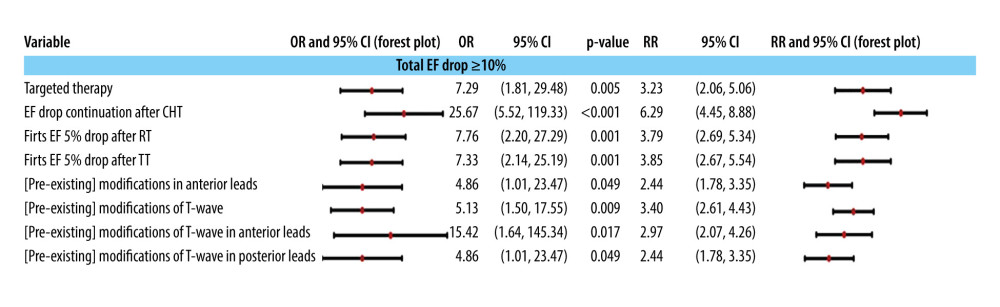
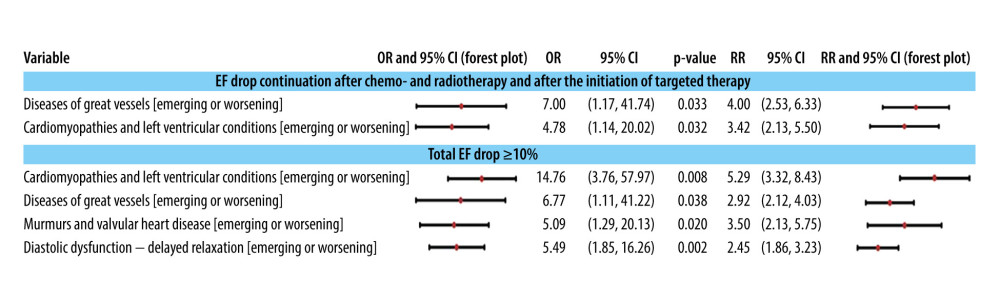
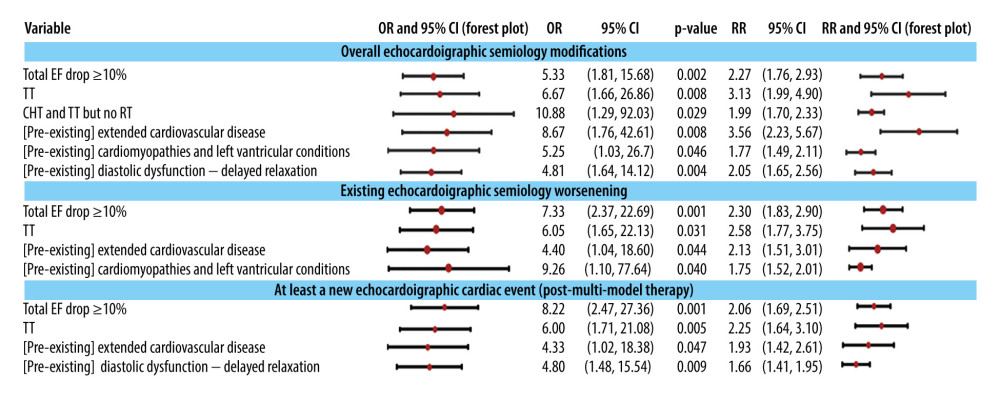
Tables
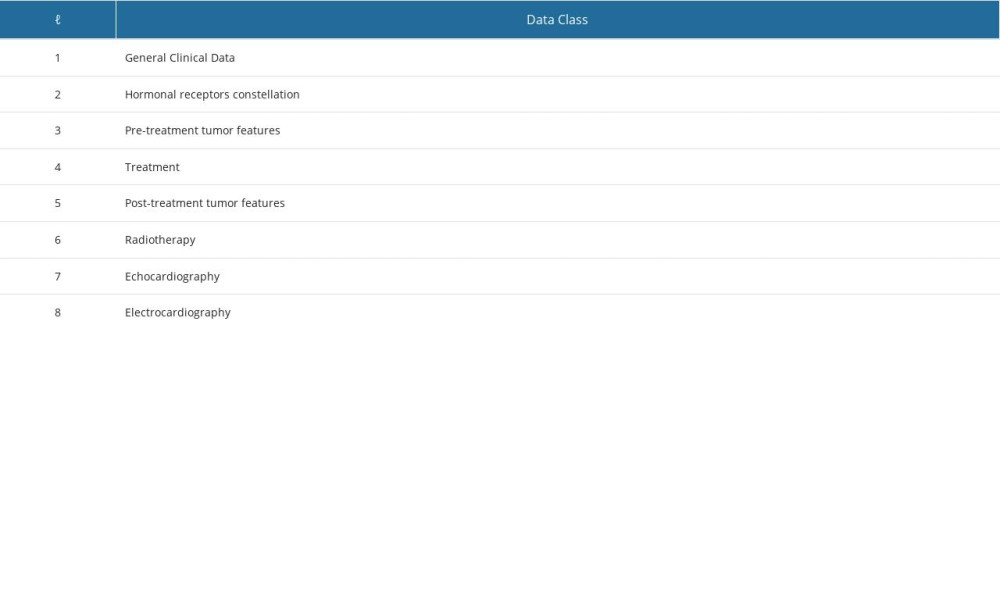
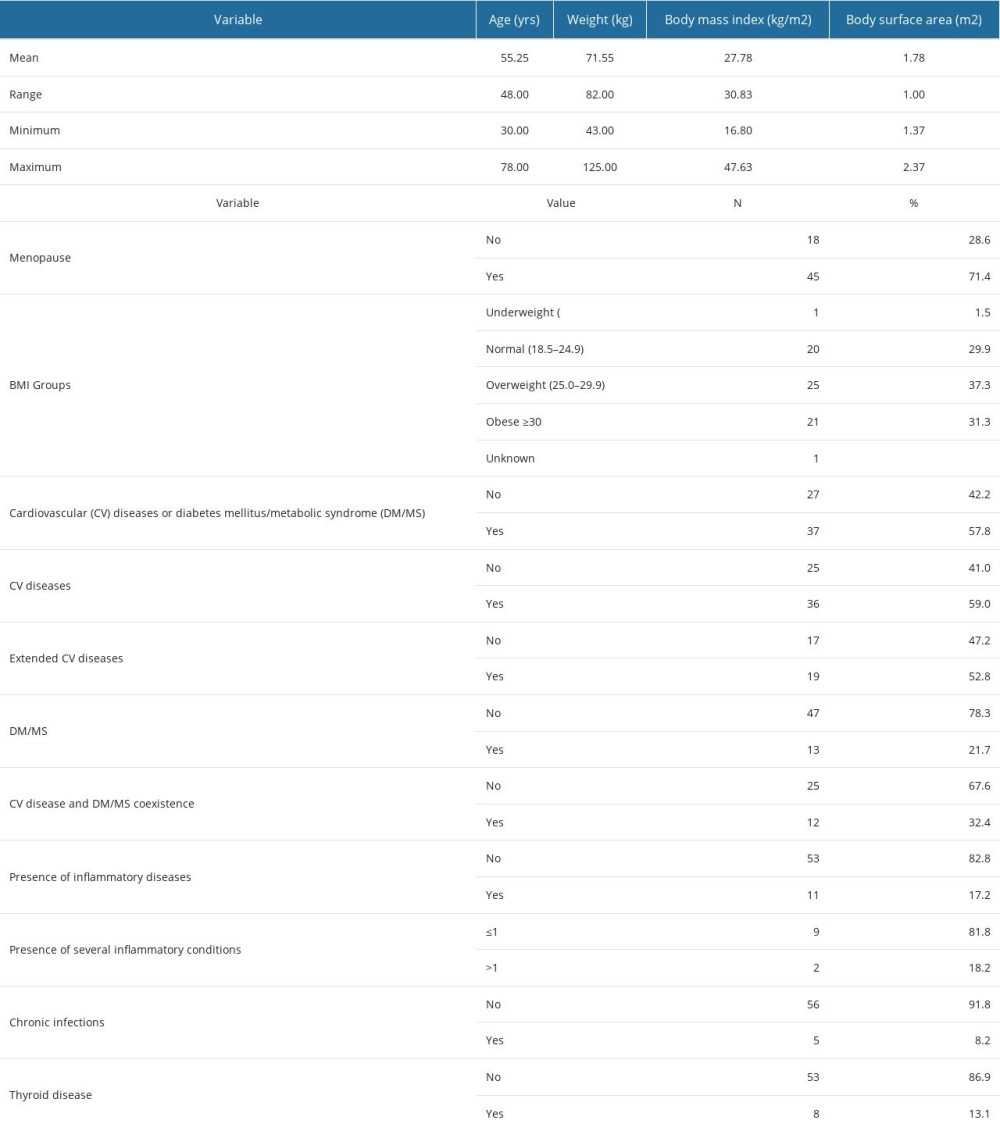
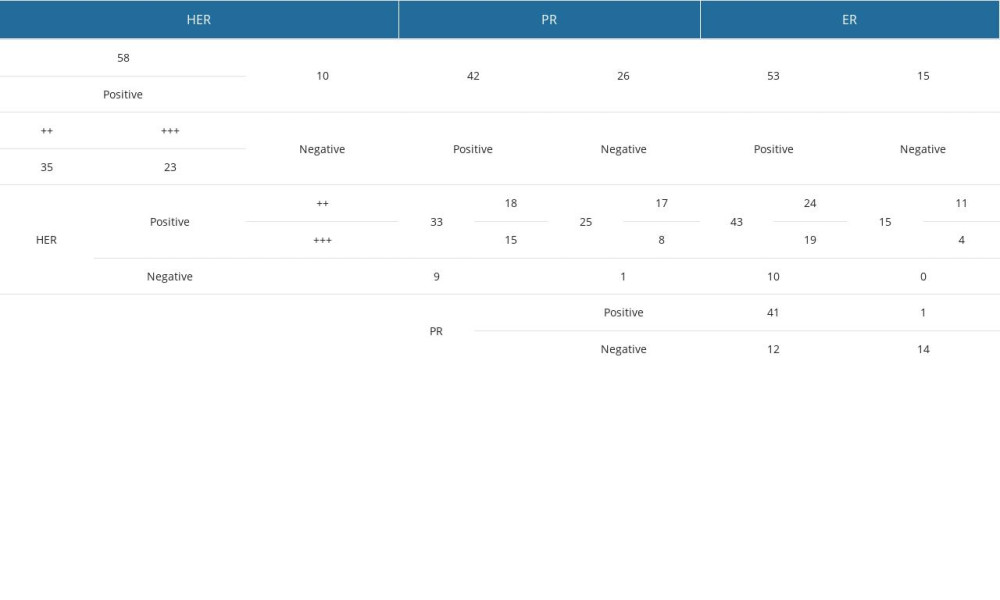
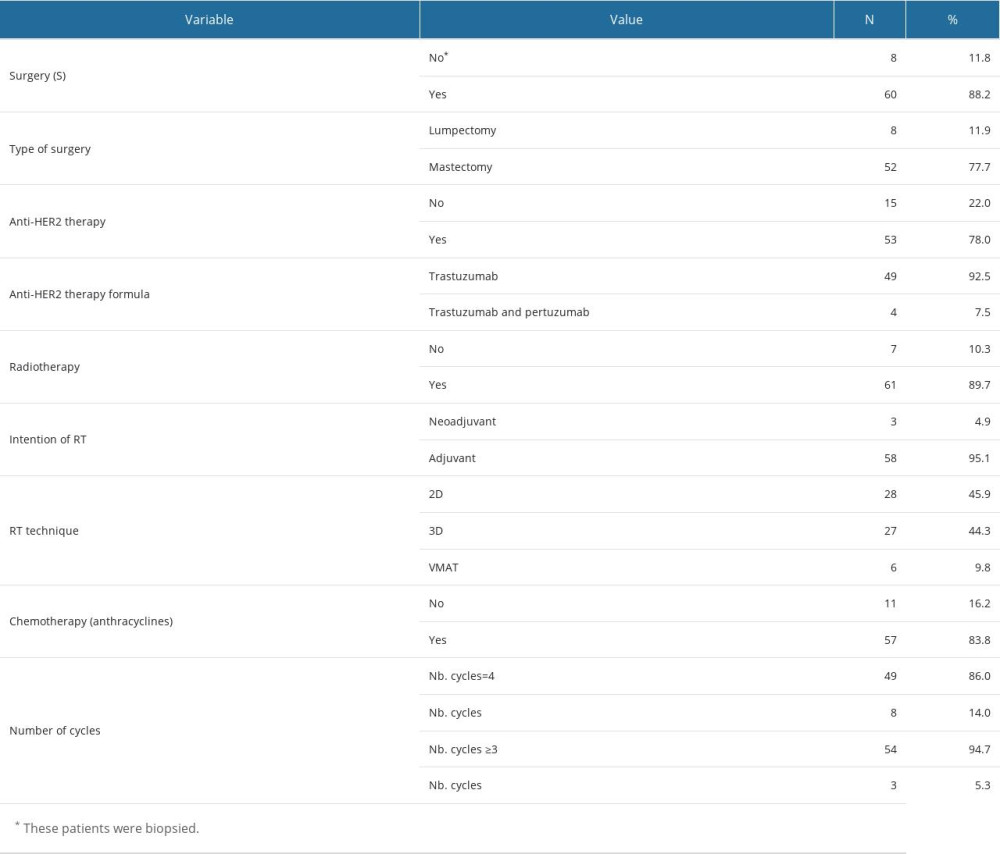
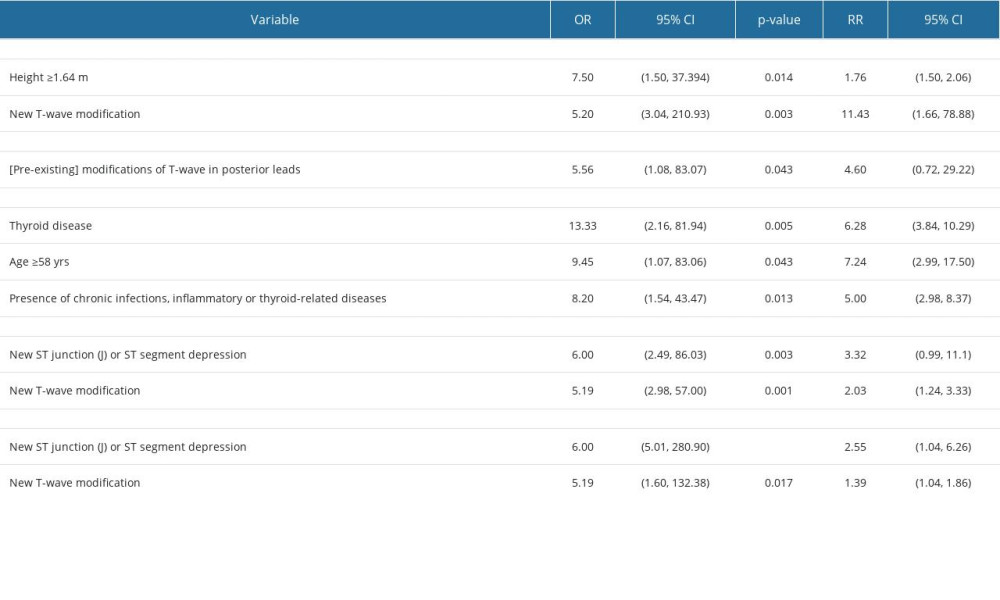
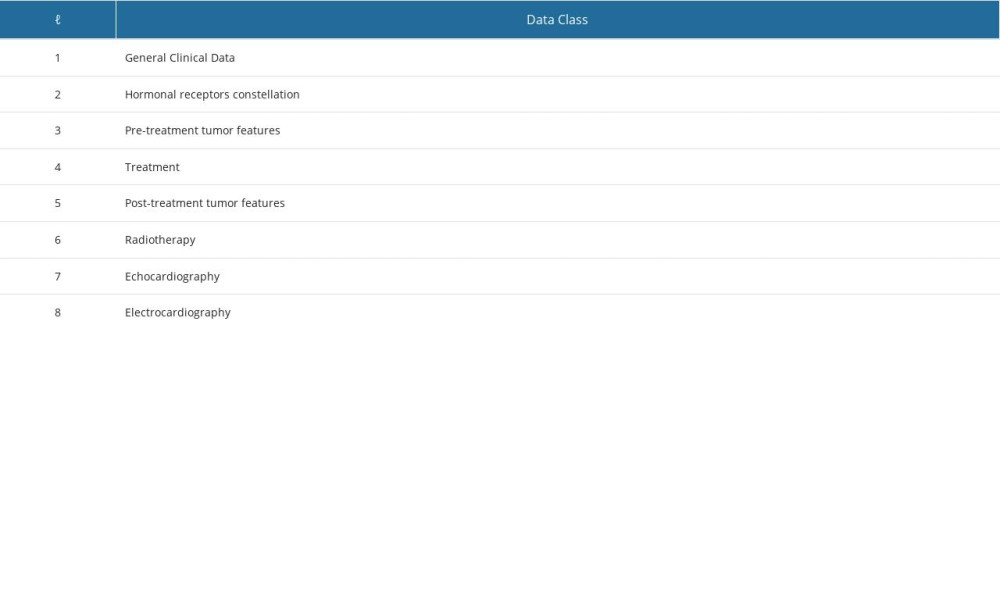
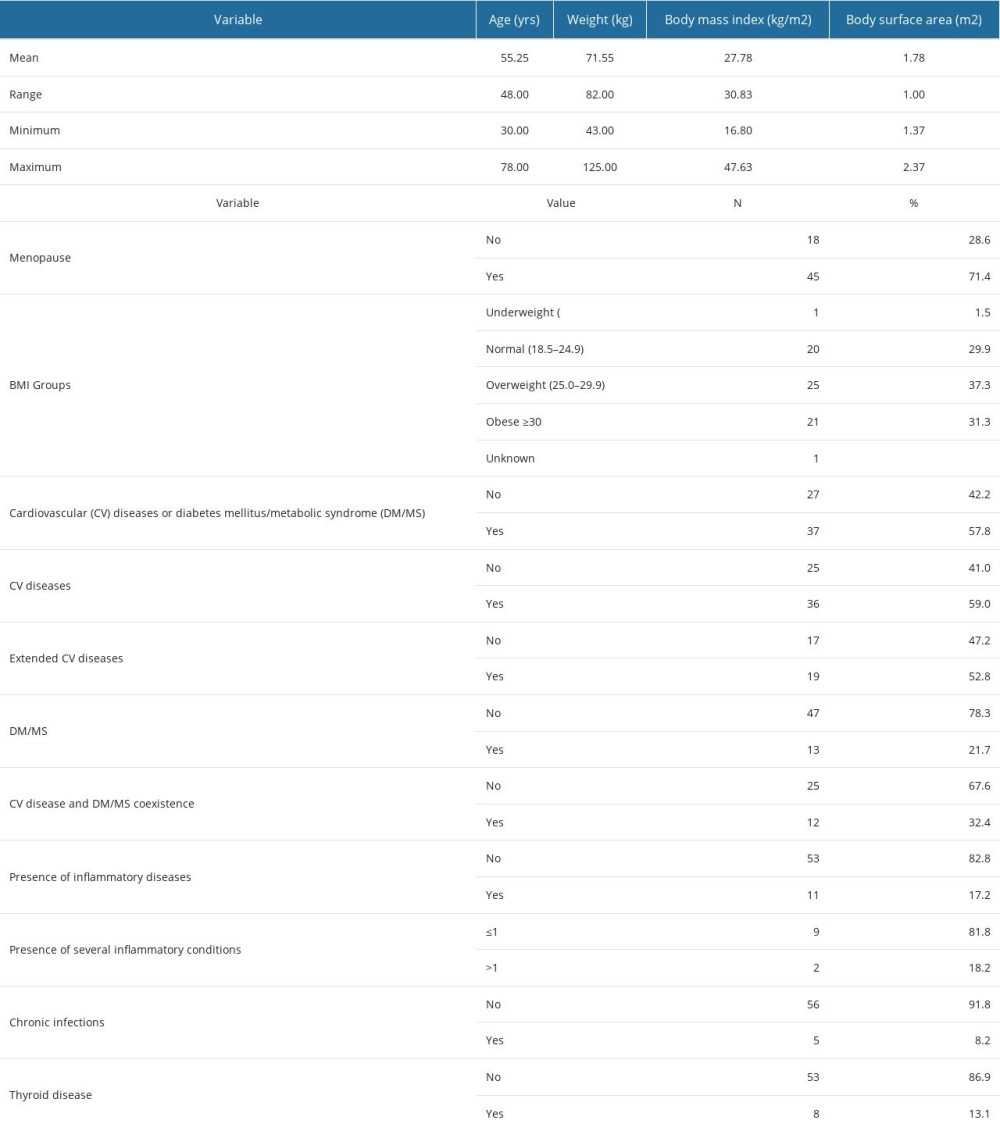
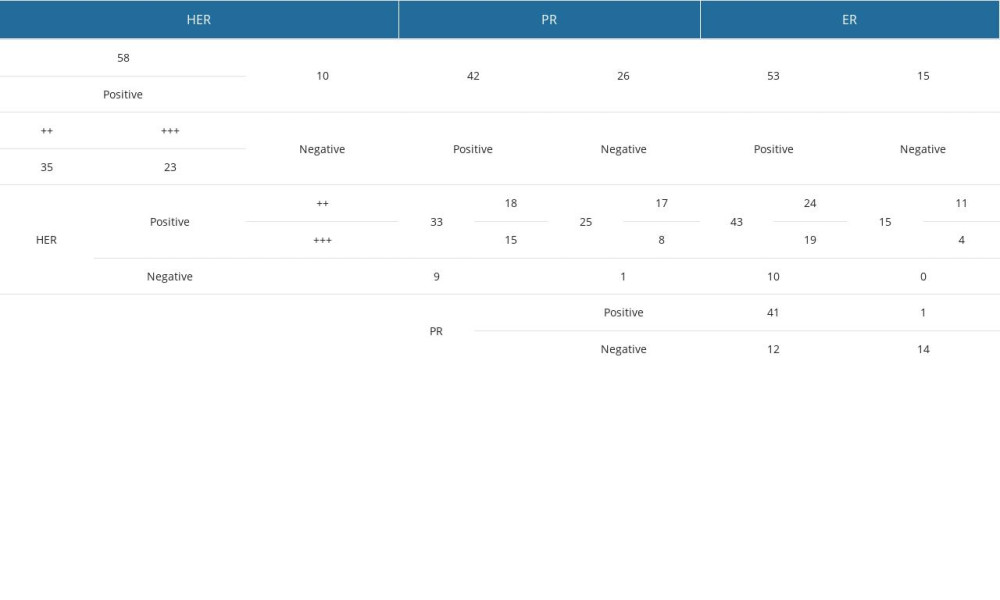
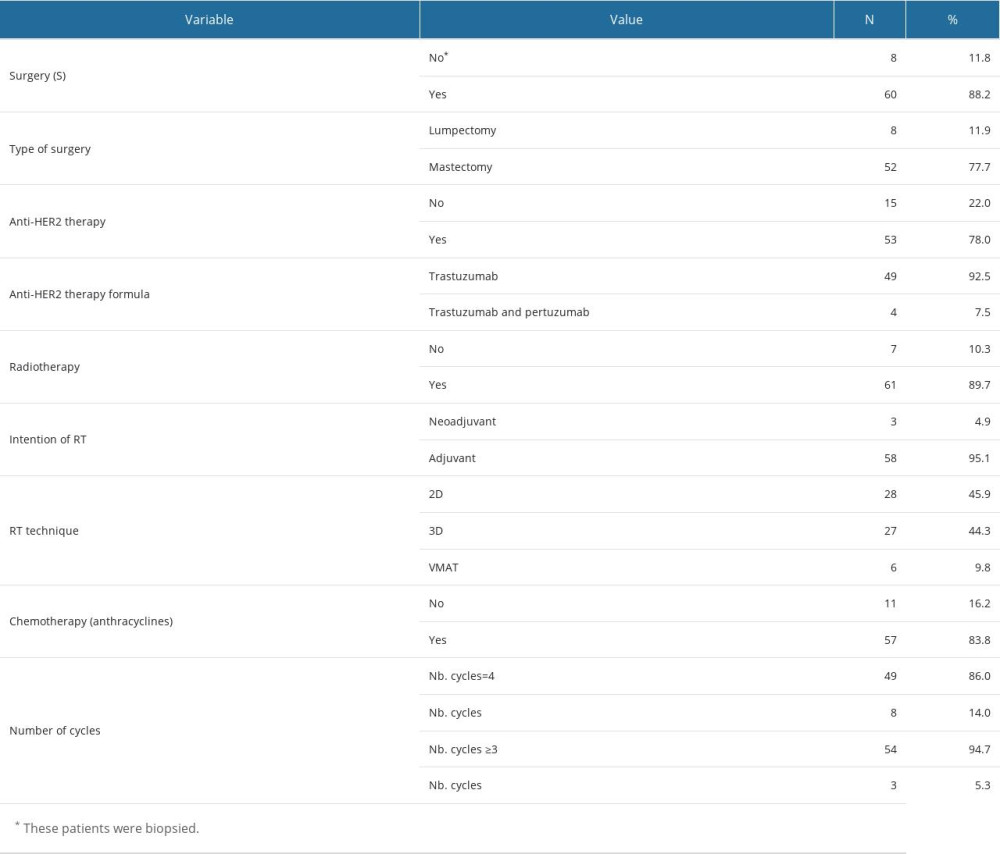
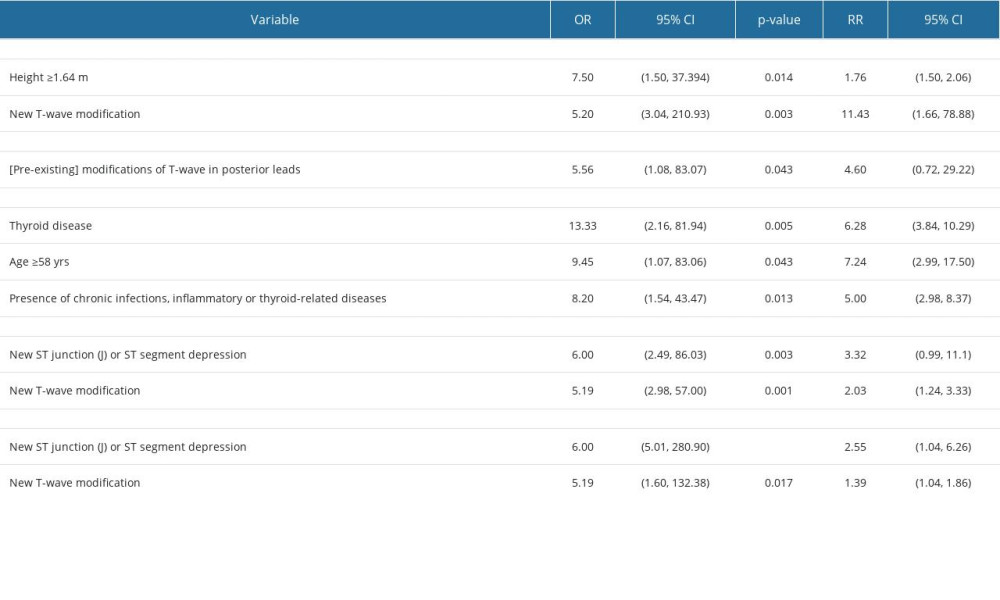
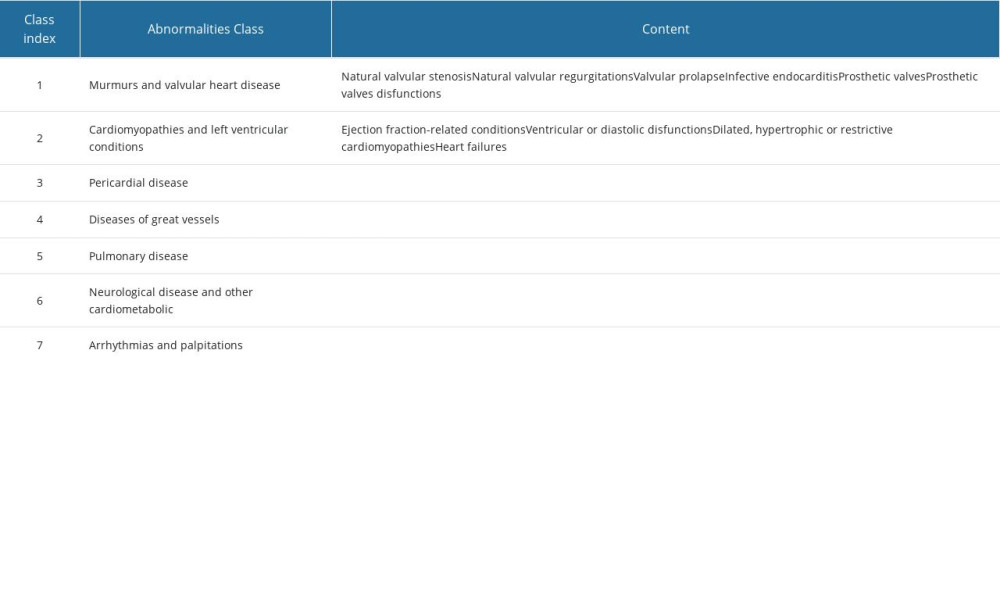
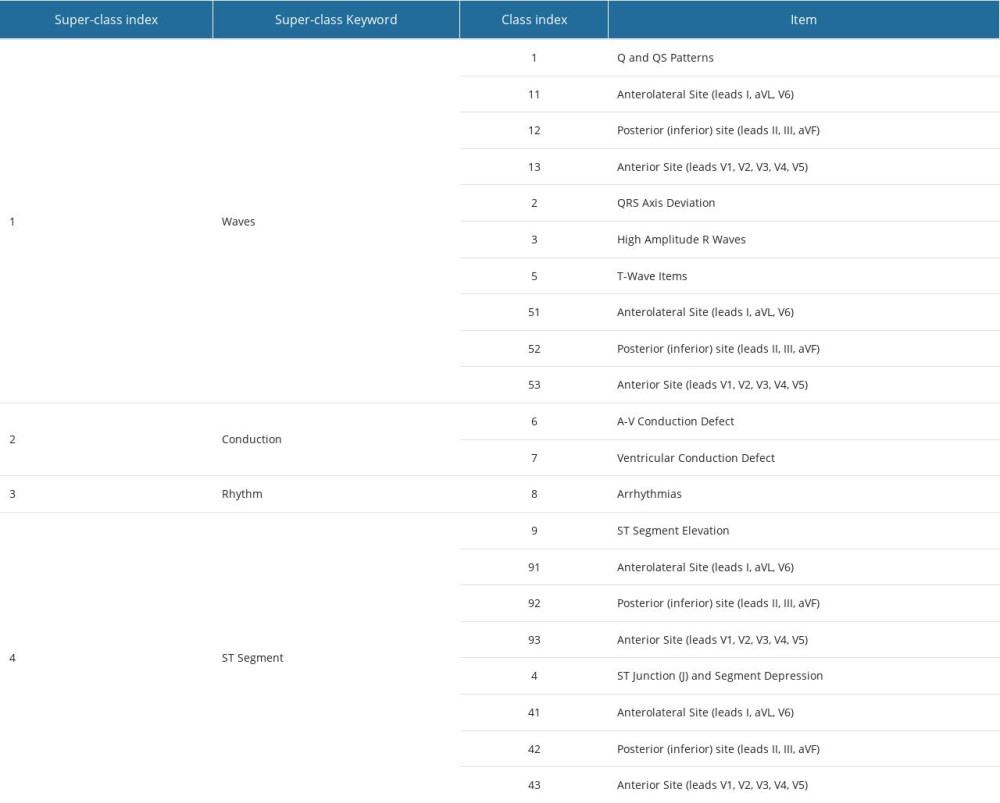
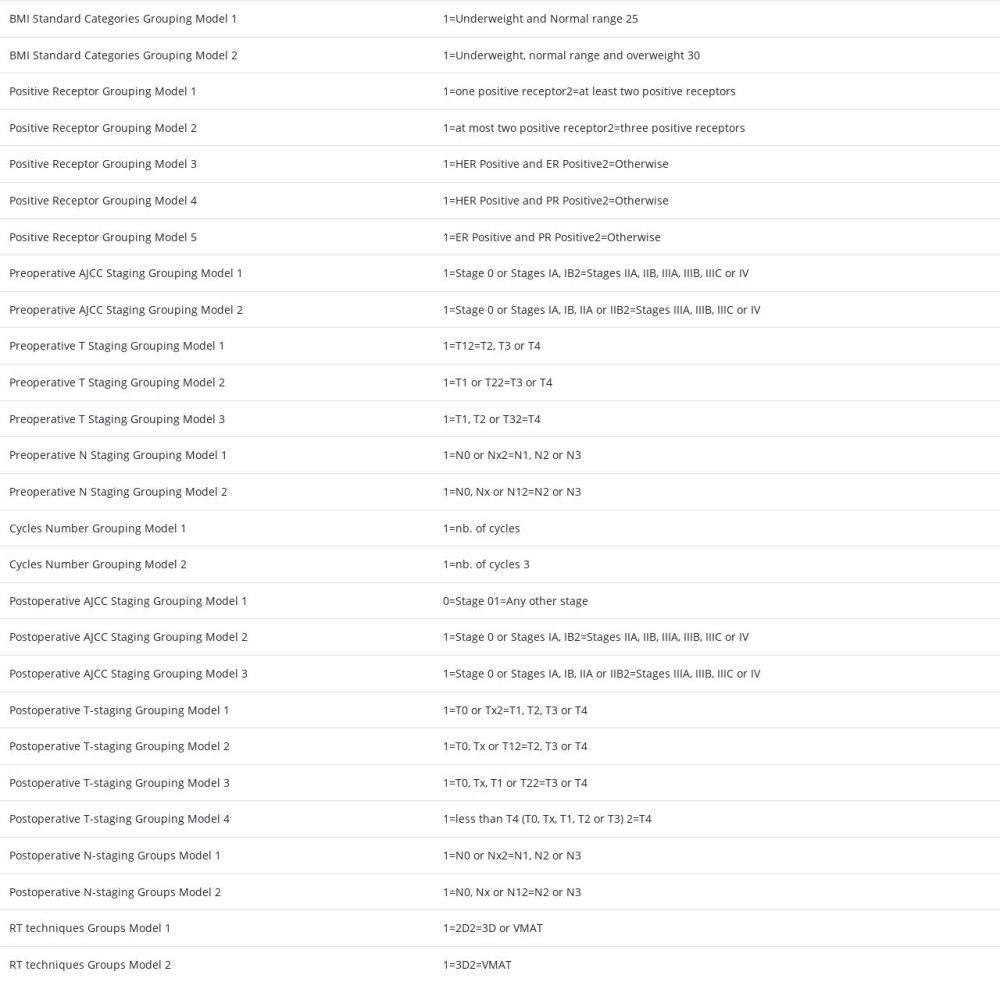
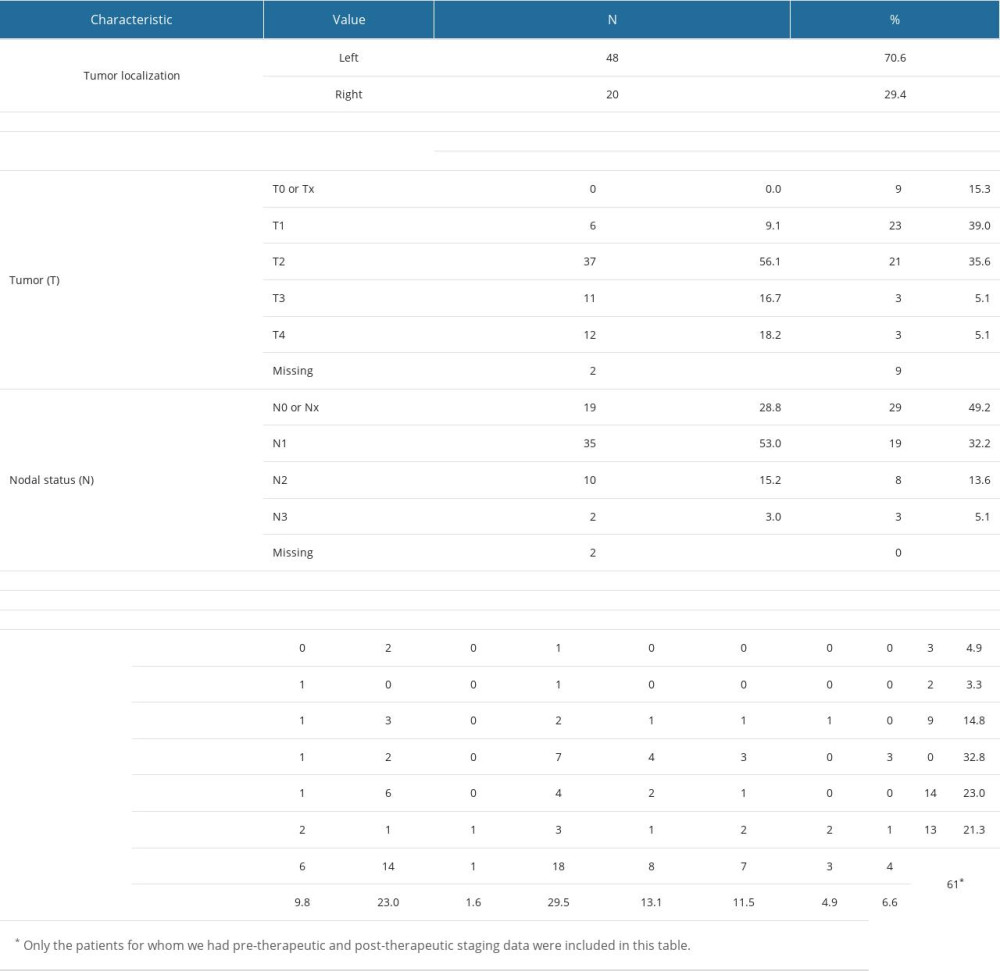
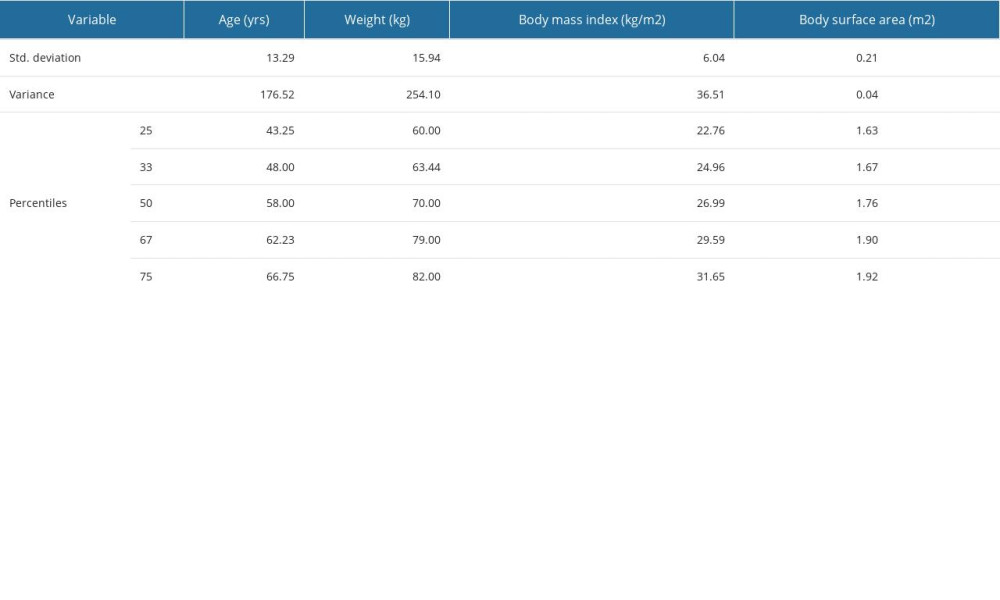
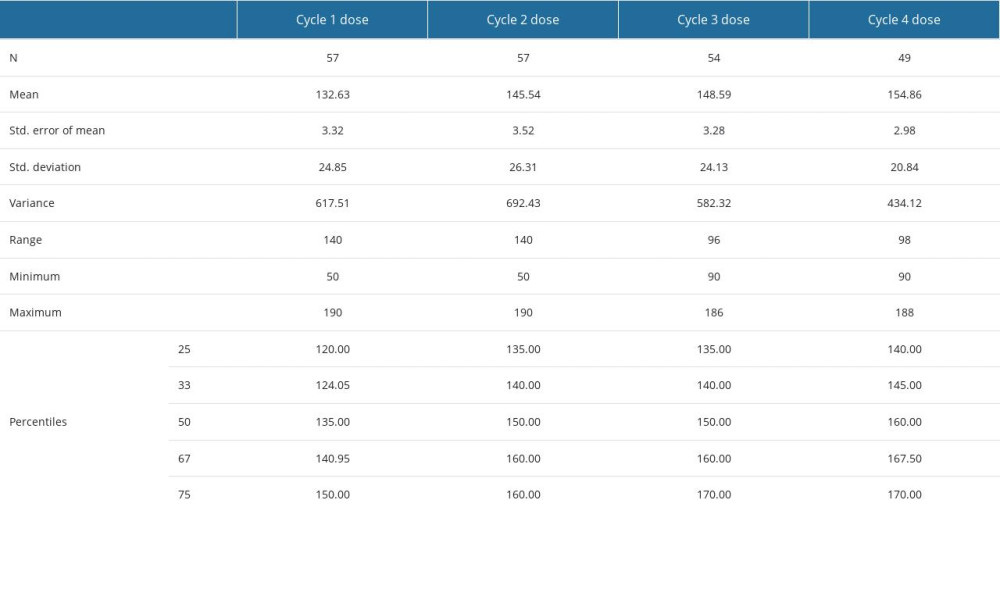
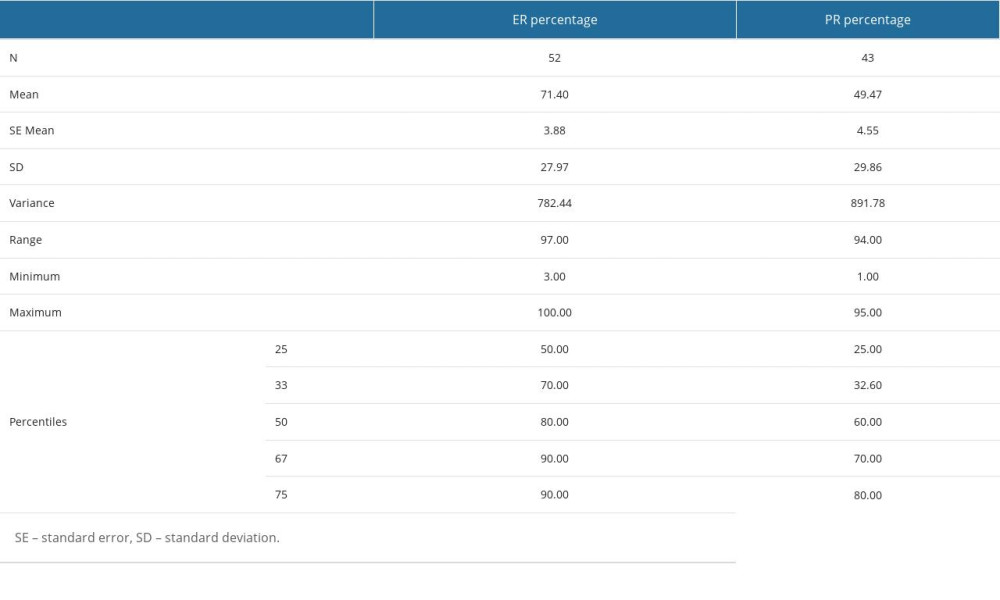
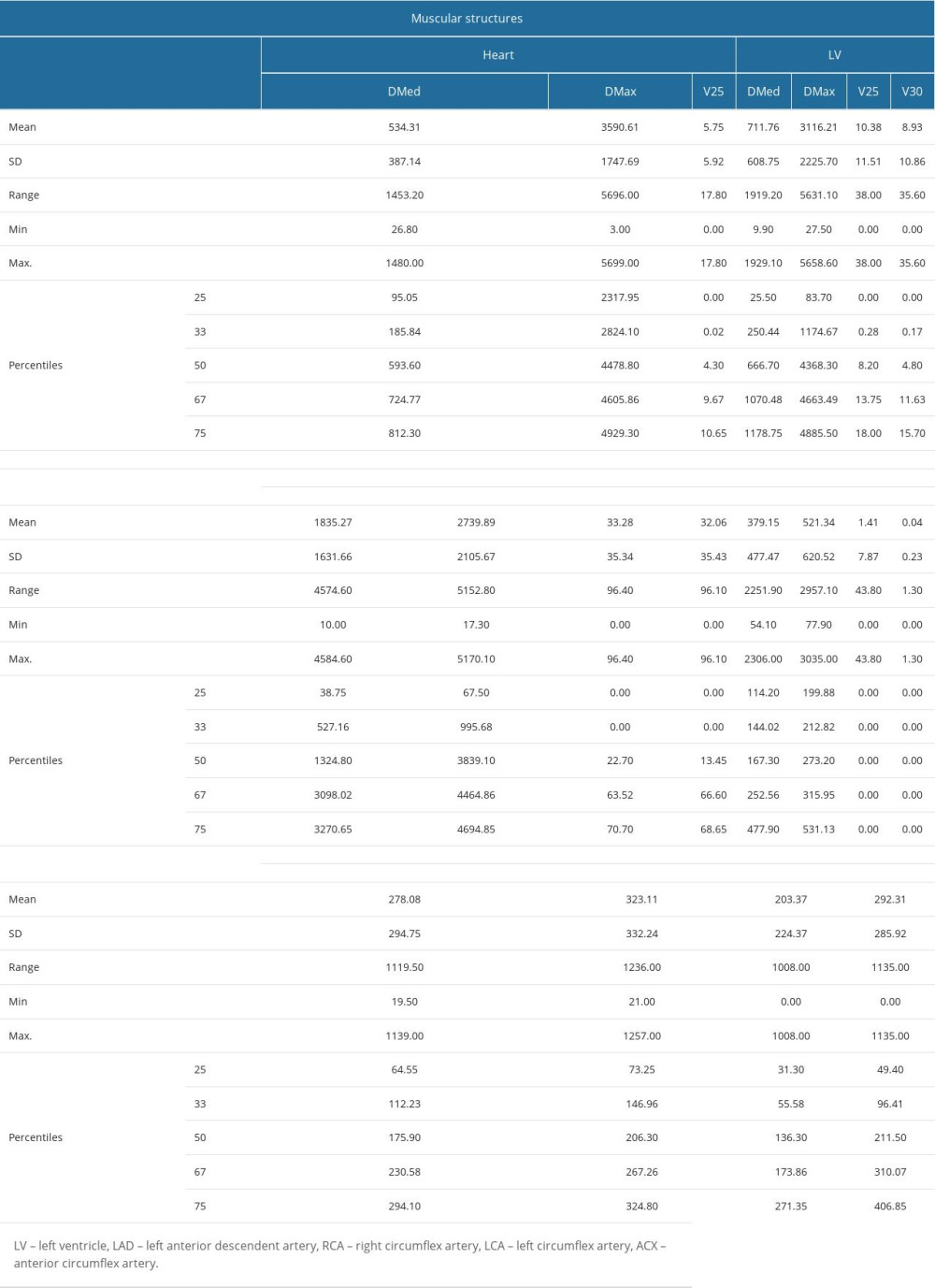
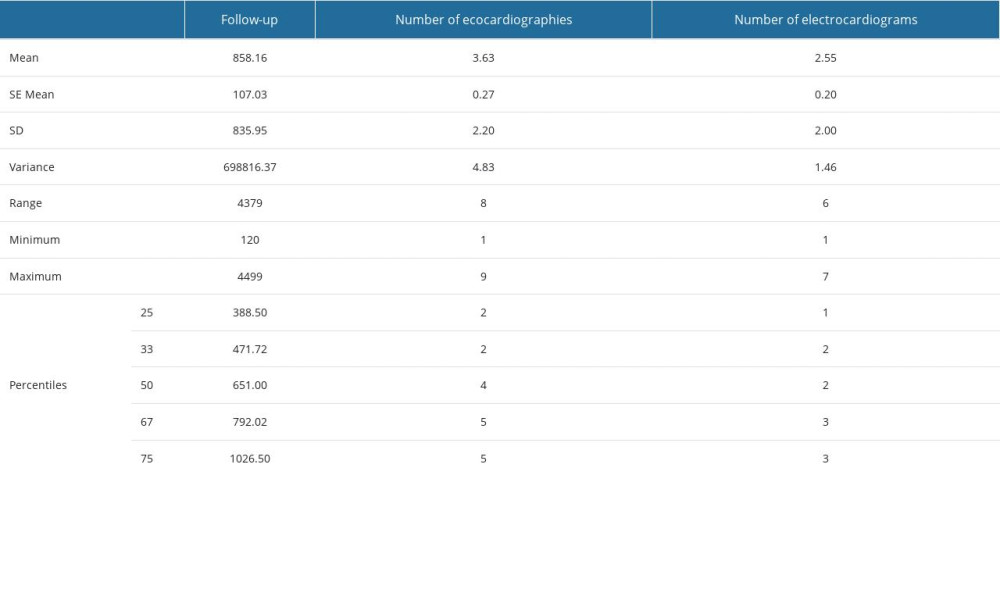
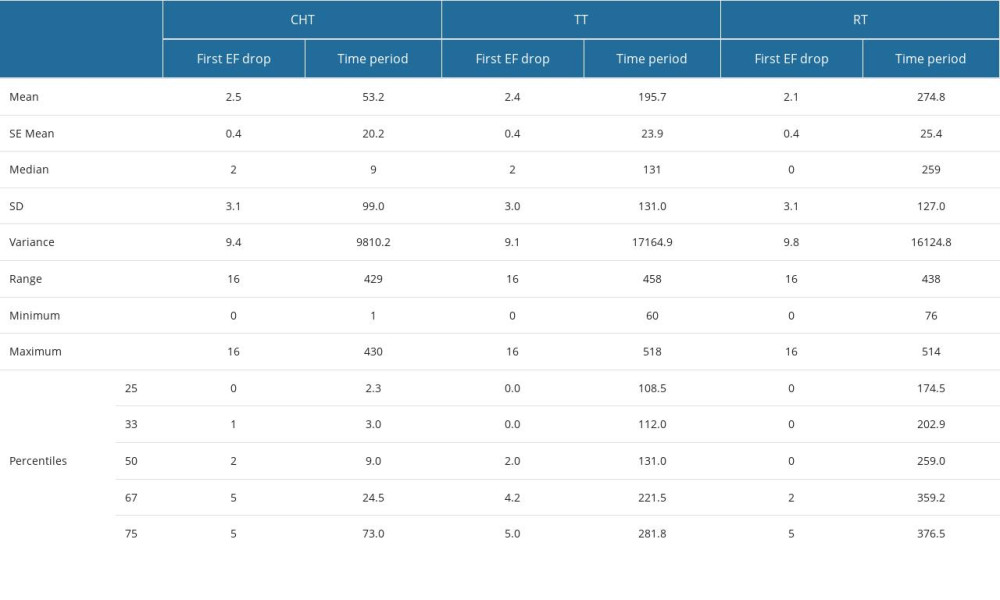
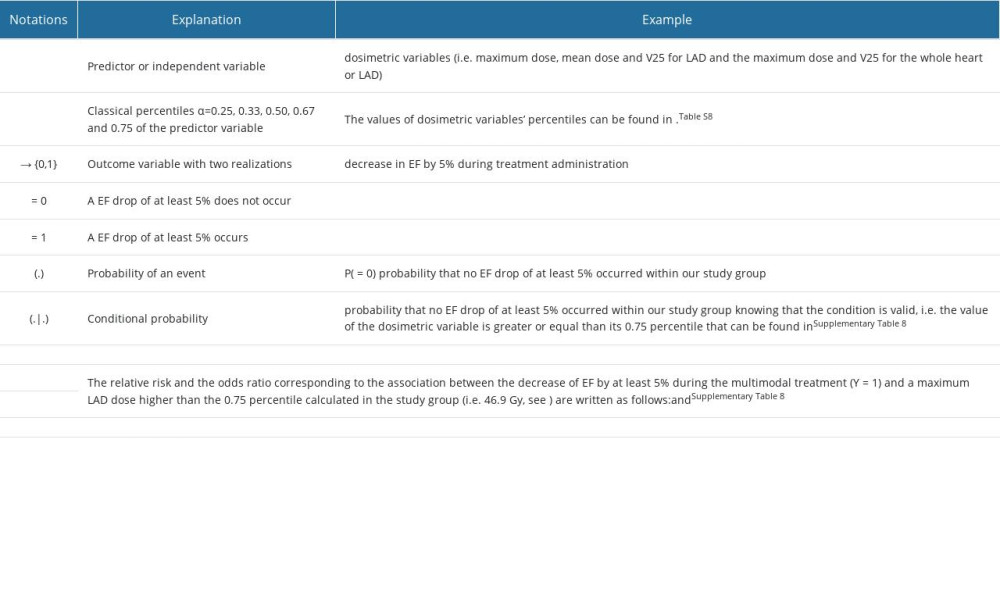
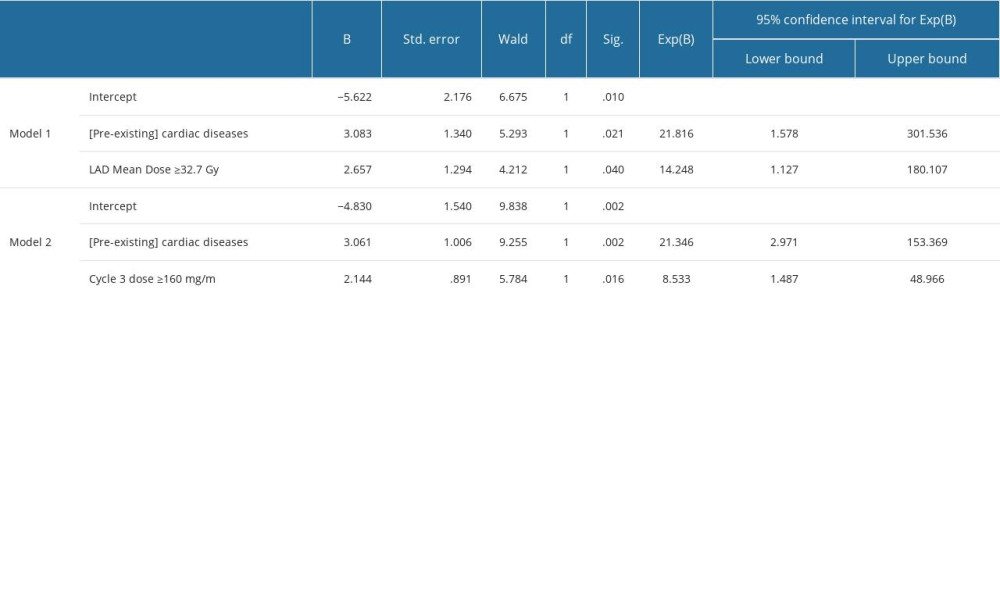
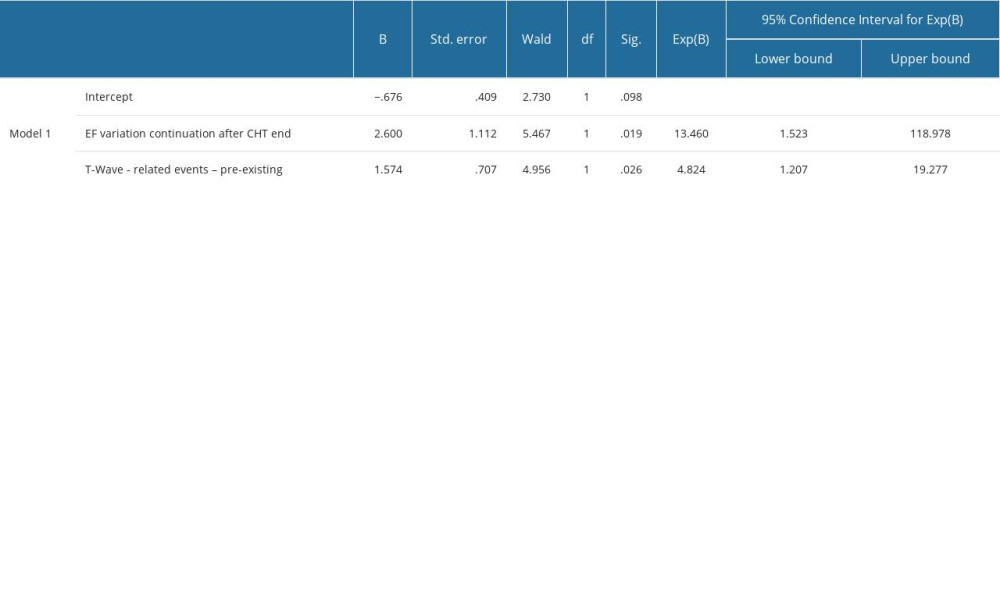
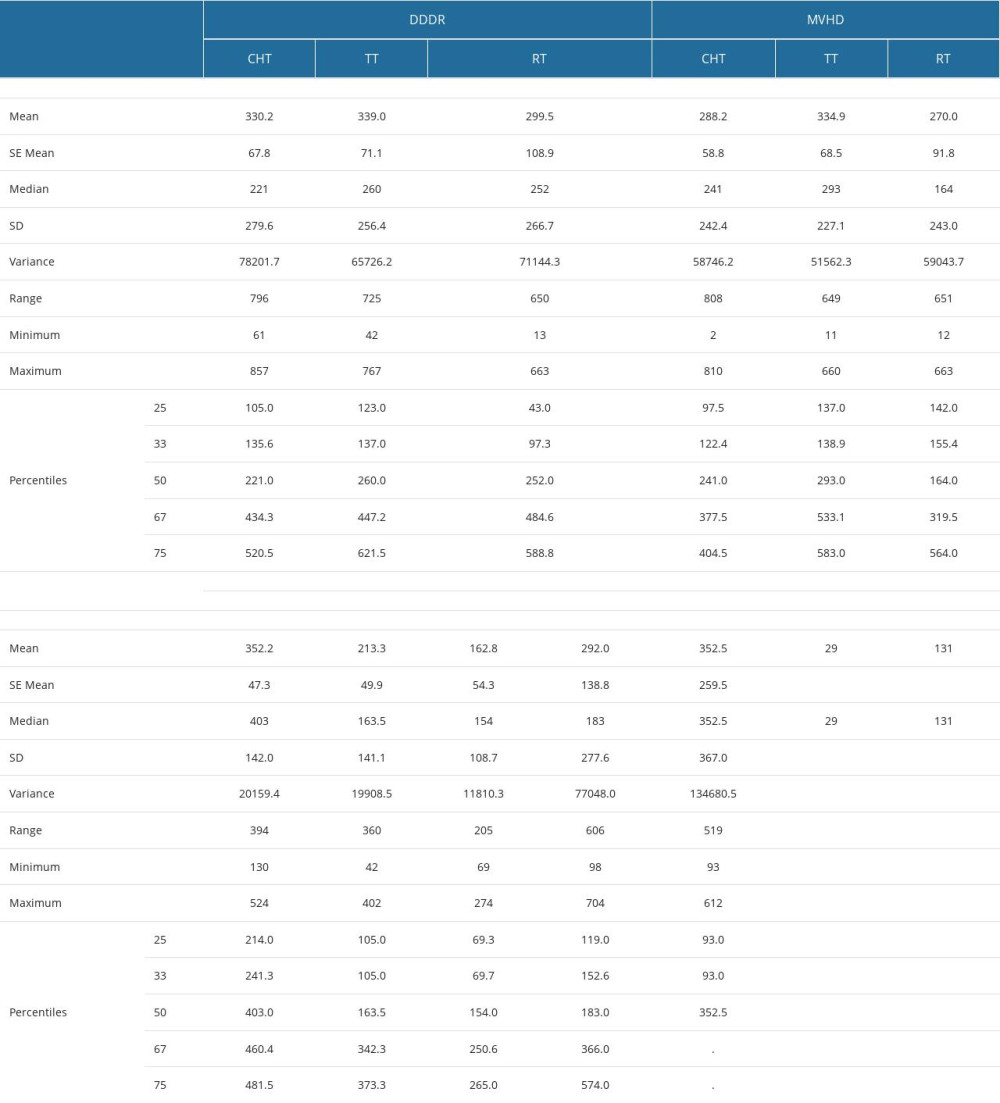
In Press
08 Mar 2024 : Animal Research
Modification of Experimental Model of Necrotizing Enterocolitis (NEC) in Rat Pups by Single Exposure to Hyp...Med Sci Monit In Press; DOI: 10.12659/MSM.943443
18 Apr 2024 : Clinical Research
Comparative Analysis of Open and Closed Sphincterotomy for the Treatment of Chronic Anal Fissure: Safety an...Med Sci Monit In Press; DOI: 10.12659/MSM.944127
08 Mar 2024 : Laboratory Research
Evaluation of Retentive Strength of 50 Endodontically-Treated Single-Rooted Mandibular Second Premolars Res...Med Sci Monit In Press; DOI: 10.12659/MSM.944110
11 Mar 2024 : Clinical Research
Comparison of Effects of Sugammadex and Neostigmine on Postoperative Neuromuscular Blockade Recovery in Pat...Med Sci Monit In Press; DOI: 10.12659/MSM.942773
Most Viewed Current Articles
17 Jan 2024 : Review article
Vaccination Guidelines for Pregnant Women: Addressing COVID-19 and the Omicron VariantDOI :10.12659/MSM.942799
Med Sci Monit 2024; 30:e942799
14 Dec 2022 : Clinical Research
Prevalence and Variability of Allergen-Specific Immunoglobulin E in Patients with Elevated Tryptase LevelsDOI :10.12659/MSM.937990
Med Sci Monit 2022; 28:e937990
16 May 2023 : Clinical Research
Electrophysiological Testing for an Auditory Processing Disorder and Reading Performance in 54 School Stude...DOI :10.12659/MSM.940387
Med Sci Monit 2023; 29:e940387
01 Jan 2022 : Editorial
Editorial: Current Status of Oral Antiviral Drug Treatments for SARS-CoV-2 Infection in Non-Hospitalized Pa...DOI :10.12659/MSM.935952
Med Sci Monit 2022; 28:e935952